Higher glucagon-to-insulin ratio is associated with elevated glycated hemoglobin levels in type 2 diabetes patients
Article information
Abstract
Background/Aims
The importance of α-cell dysfunction in the pathogenesis of type 2 diabetes has re-emerged recently. However, data on whether relative glucagon excess is present in clinical settings are scarce. We aimed to investigate associations between glucagon-to-insulin ratio and various metabolic parameters.
Methods
A total of 451 patients with type 2 diabetes naïve to insulin treatment were recruited. Using glucagon-to-insulin ratio, we divided subjects into quartiles according to both fasting and postprandial glucagon-to-insulin ratios.
Results
The mean age of the subjects was 58 years, with a mean body mass index of 25 kg/m2 The patients in the highest quartile of glucagon-to-insulin ratio had higher glycated hemoglobin (HbA1c) levels. HbA1c levels were positively correlated with both fasting and postprandial glucagon-to-insulin ratios. Subjects in the highest quartile of postprandial glucagon-to-insulin ratio were more likely to exhibit uncontrolled hyperglycemia, even after adjusting for confounding factors (odds ratio, 2.730; 95% confidence interval, 1.236 to 6.028; p for trend < 0.01).
Conclusions
Hyperglucagonemia relative to insulin could contribute to uncontrolled hyperglycemia in type 2 diabetes patients.
INTRODUCTION
Defects in insulin secretion and aggravation of insulin resistance are considered the primary factors affecting diabetes development and progression. However, apart from insulin itself, various other factors may also affect glucose regulation in diabetes [1,2]. One of these factors may be the dysregulation of glucagon upon pancreatic α-cell dysfunction [3-5]. By enhancing gluconeogenesis in the liver, glucagon levels are elevated upon fasting to prevent hypoglycemia [6-8]. The secretory mechanism of glucagon from α-cells has been proposed to be regulated by insulin concentrations from β-cells, which are located closely to α-cells [9,10]. From a theoretical view, when insulin secretion increases after meals, glucagon secretion must be suppressed [11-13]. However, this mechanism appears to be dysregulated in diabetic patients who show relatively higher postprandial glucagon levels than normal subjects [14,15]. This suggests that α-cell resistance or dysfunction may be present in patients with diabetes [4,5,16].
In this aspect, Unger [17] has suggested the ‘bihormonal-abnormality’ hypothesis in regards to the development of diabetes, stating that both relative or absolute hyperglucagonemia and insulin deficiency may be present in diabetic subjects. Recently, both insulin and glucagon have received attention as critical controllers of blood glucose levels [18-21]. Interestingly, in patients with pancreatic cancer, glucagon-to-insulin ratio after a 75-g oral glucose challenge was independently correlated with glycated hemoglobin (HbA1c) levels [22]. These results suggested that relative hyperglucagonemia might influence hyperglycemia in diabetes and that modulating the activity of glucagon might be a promising target for achieving glycemic control in a subgroup of diabetic patients [23,24]. Nevertheless, data on increases in glucagon relative to insulin and their associations with other clinical parameters are scarce.
In this study, we evaluated whether increases in glucagon relative to insulin (glucagon-to-insulin ratio) are associated with various metabolic parameters and assessed the contributions of relative hyperglucagonemia on blood glucose control.
METHODS
Study design and population
Type 2 diabetes mellitus (DM) patients who visited the outpatient clinic at the endocrinology department of Gangnam Severance Hospital in Seoul, Korea from March 2012 to January 2013 were registered (n = 533; 342 men, 191 women). The study subjects underwent routine blood tests to assess insulin and glucagon levels both before and 2 hours after a meal. This study was approved by the local Institutional Review Board (IRB approval number: 3-2014-0169). Subjects with gestational diabetes or type 1 DM or who had been treated with insulin were excluded from the study. We divided the study population (n = 451; 308 men, 143 women) into quartiles of the same number of patients according to both fasting and postprandial glucagon-to-insulin ratios, respectively.
Biochemical measurement
Plasma glucose was measured by the glucose oxidase method using a 747 Automatic Analyzer (Hitachi, Tokyo, Japan). The levels of total cholesterol, high density lipoprotein cholesterol (HDL-C), triglycerides, and alanine aminotransferase (ALT) were evaluated by an enzymatic colorimetric method (Hitachi 747, Daiichi, Tokyo, Japan). Low density lipoprotein cholesterol content was calculated according to the Friedewald formula [25]. HbA1c levels were measured by high-performance liquid chromatography (Cobas Integra 800, Roche, Mannheim, Germany). Insulin and glucagon levels were measured using a radioimmunoassay method (Roche Diagnostics for insulin; MP Biomedicals, Irvine, CA, USA for glucagon).
Statistical analysis
Continuous variables are presented as the mean ± standard deviation (SD) and categorical variables as absolute numbers (percentage). The significance of differences in glucagon-to-insulin ratio quartiles was analyzed using either the one-way analysis of variance, the chi-square test, or Fisher exact test as appropriate. The degree of correlation between metabolic parameters and glucagon-to-insulin ratio was presented by Pearson correlation coefficient (r). Independent predictors of gluca gon-to-insulin ratio were assessed by multiple stepwise linear regression analyses and logistic regression models were used to determine the odds ratio (OR) of the covariates. All statistical analyses were performed using SPSS version 20.0 (IBM Co., Armonk, NY, USA), and p values < 0.05 were considered statistically significant.
Ethical standard
This human study has been reviewed by the appropriate ethics committee and has therefore been performed in accordance with the ethical standards stipulated in the 1964 Declaration of Helsinki and its following amendments.
Human and animal rights disclosure
All procedures followed were in accordance with the ethical standards of the responsible committee on human experimentation (institutional and national) and with the Helsinki Declaration of 1975, as revised in 2008.
RESULTS
Clinical and biochemical characteristics of the study population
The mean age of the whole study population was 57.7 ± 12.7 years, and the mean duration of diabetes was 8.7 ± 7.8 years. The mean HbA1c level was 6.96% ± 1.20% (52.53 ± 13.13 mmol/mol), and the mean body mass index (BMI) was 24.98 ± 3.29 kg/m2. Patients in the highest quartiles of both fasting and postprandial glucagon-to-insulin ratios had the highest HbA1c levels (7.19% ± 1.47%, p = 0.02 for fasting; and 7.28% ± 1.57%, p < 0.01 for postprandial glucagon-to-insulin ratio, respectively) and tended to have the longest duration of diabetes (10.0 ± 8.1 years, p = 0.07 for fasting; and 11.0 ± 9.2 years, p < 0.01 for postprandial glucagon-to-insulin ratio, respectively). These patients also had lower BMI and ALT levels, as well as a higher HDL-C levels, than the other groups (Tables 1 and 2).
Association between metabolic parameters and glucagon-to-insulin ratio
Correlations between metabolic parameters and fasting or postprandial glucagon-to-insulin ratio were analyzed. Both fasting and postprandial glucose, HbA1c and HDL-C levels were positively correlated with fasting glucagon-to-insulin ratio, whereas BMI, triglycerides, and ALT levels showed negative correlations. Duration of diabetes, HbA1c, and HDL-C were positively correlated with postprandial glucagon-to-insulin ratio; BMI, triglycerides, and ALT showed a negative correlation with postprandial glucagon-to-insulin ratio (Table 3).
Independent factors associated with fasting and postprandial glucagon-to-insulin ratios
We analyzed independent factors associated with both fasting and postprandial glucagon-to-insulin ratios. HbA1c, BMI, and ALT were independent factors associated with fasting glucagon-to-insulin ratio. The postprandial glucagon-to-insulin ratio was significantly and independently associated with HbA1c, duration of diabetes, BMI, and ALT. In both fasting and postprandial states, elevation of HbA1c levels was independently associated with higher fasting and postprandial glucagon-to-insulin ratios (regression coefficient = 1.92, standardized error = 0.43, p < 0.01 for fasting; and regression coefficient = 0.38, standardized error = 0.11, p < 0.01 for postprandial glucagon-to-insulin ratio) (Table 4).
Association of glucagon-to-insulin ratio with the risk of uncontrolled blood glucose
To assess the risk of uncontrolled blood glucose levels with respect to glucagon-to-insulin ratio, we performed multiple logistic regression analysis. After adjusting for age, sex, BMI, HDL-C, and ALT, subjects in the highest quartile of postprandial glucagon-to-insulin ratio were 3.7-fold more likely to have uncontrolled diabetes, defined by HbA1c ≥ 7.5% (58.5 mmol/mol) (OR, 3.681; 95% confidence interval [CI], 1.752 to 7.736; p for trend < 0.01). Moreover, subjects in the highest quartile of postprandial glucagon-to-insulin ratio were 2.7-fold more likely to have a higher HbA1c level, even after further adjusting for the duration of diabetes and the use of metformin, sulfonylurea, and dipeptidyl peptidase-4 inhibitor (DPP-4 I) (OR, 2.730; 95% CI, 1.236 to 6.028; p for trend < 0.01) (Table 5). In a model adjusting for age, sex, BMI, HDL-C, ALT, duration of diabetes, and use of metformin, sulfonylurea, and DPP-4 I, ORs for uncontrolled diabetes increased gradually with higher quartiles of postprandial glucagon-to-insulin ratio (OR, 0.739; 95% CI, 0.321 to 1.701 for Quartile 2; OR, 2.041; 95% CI, 0.946 to 4.405 for Quartile 3; and OR, 2.730; 95% CI, 1.236 to 6.028 for Quartile 4, respectively) (Table 5, Fig. 1).
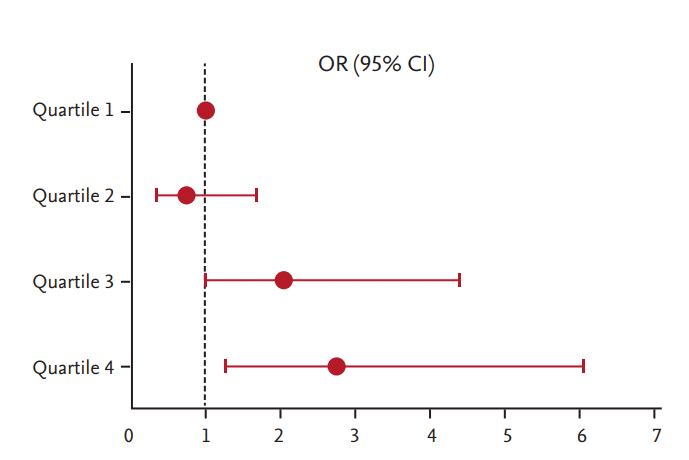
Odds ratio for glycated hemoglobin ≥ 7.5% according to postprandial glucagon-to-insulin ratio quartiles. Logistic regression was used for calculating odds ratios (ORs) with 95% confidence intervals (CIs). The reference group comprised patients in the lowest quartile of postprandial glucagon-to-insulin ratio (Quartile 1). Adjusted for age, sex, body mass index, high density lipoprotein cholesterol, alanine aminotransferase, duration of diabetes, and metformin, sulfonylurea, and dipeptidyl peptidase-4 inhibitor use.
DISCUSSION
In this study, we found that patients with a higher glucagon-to-insulin ratio upon fasting or in a postprandial state exhibit worse blood glucose control (i.e., higher HbA1c levels). Both fasting and postprandial glucagon-to-insulin ratios were found to be positively correlated with HbA1c and HDL-C levels, whereas they were negatively correlated with ALT. Interestingly, ORs for higher HbA1c levels increased gradually with increasing quartiles of postprandial glucagon-to-insulin ratio, demonstrating that glucagon-to-insulin ratio reflects persistent, but gradual, degrees of glycemic control across the whole DM population, rather than there being an abrupt cut-off value of glucagon that indicates glycemic control in an all-or-none fashion. Our results suggest that an increased level of glucagon, relative to insulin, is closely associated with poor glycemic control.
Our study highlighted glucagon excess relative to insulin in a postprandial state as being associated with poor glycemic control. Previously, several studies suggested that absolute or relative hyperglucagonemia may be a cause of poor glycemic control in subjects with impaired glucose tolerance or diabetes [13,16,26-30]. However, most of these studies were performed in a small number of subjects, and no study has explored what features are associated with relative glucagon excess [11,16,28,31,32]. Overcoming these limitations, we have demonstrated in a relatively large number of subjects (n = 451) that relative glucagon excess is associated with various metabolic parameters in clinical practice.
We initially hypothesized that subjects with relative glucagon excess might be associated with poor glycemic control, together with both insulin resistance and worse metabolic parameters [11,15,33,34]. Interestingly, we found that subjects with higher glucagon-to-insulin ratio had lower BMI and higher HDL despite poor glycemic control (higher HbA1c levels). The characteristics of our study group seem to be in line with those of non-obese Asian diabetic patients with islet dysfunction [30,35]. A previous study showed that lean patients with type 2 diabetes can exhibit better lipid profile than obese patients, even though the lean patients have more uncontrolled hyperglycemia [36]. Lower BMI is normally accompanied with better metabolic profile [37]. However, in non-obese patients with type 2 diabetes, BMI with insulin resistance is not the major determinant of glycemic control [38]. Thus, there could have been a discrepancy between metabolic profiles and glycemic control in our study population [36]. In these individuals, β-cell dysfunction, reflected as insulin deficiency, rather than insulin resistance, may be the dominant factor for uncontrolled hyperglycemia [38]. In addition to this, we assume that not only β-cell hypofunction but also relative α-cell hyperfunction may contribute to poor glycemic control in this study population. Several papers have found glucagon excess to be an independent pathogenesis from insulin resistance in type 2 diabetes [13,39,40], and hyperglucagonemia has been continually detected in diabetic patients without obesity [31]. Additionally, a previous study found decreased β-cell and increased α-cell mass in pancreatic islets of Korean type 2 diabetes patients with a BMI less than 25 kg/m2 [30]. In the present study, we noted that glucagon-to-insulin ratio exhibited an increasing tendency as the duration of diabetes became longer. This implies that overall islet cell dysfunction becomes worse as type 2 diabetes progresses. Therefore, our study suggests that, in patients with lower BMI and long-standing type 2 diabetes, strategies to suppress glucagon excess together with promoting insulin secretion may be helpful.
The relationship between glucagon-to-insulin ratio and HbA1c has been rarely studied. Jin et al. [22] showed a linear association between glucagon-to-insulin ratio and HbA1c in subjects with pancreatic cancer, a tendency which was similar to that in our study of data for type 2 diabetic subjects. In our study, we further demonstrated that glucagon-to-insulin ratio is a significant determinant of glycemic control. The OR of postprandial glucagon-to-insulin ratio followed a significantly increasing trend in analysis of p for trend. Also, interestingly, the general shape of ORs followed a J-shape, with postprandial glucagon-to-insulin ratios in quartile 2 < quartile 1 and those in quartiles 3 and 4 > quartile 1. The possible reasons for this J-shaped association between the glycemic control and the glucagon-to-insulin ratio could be the result of unique clinical characteristics in quartile 2, such as higher age and higher DPP-4 I use, compared to the other quartiles.
This study is not without limitations. Most of the study subjects were from urban areas and had generally well-controlled glucose levels, which may not represent the entire diabetic population. This study also has the general limitation of being cross-sectional study, and thus, it cannot demonstrate whether there is a causal relationship between glucose control and glucagon-to-insulin ratio. Postprandial glucagon and insulin in this study were not measured after oral glucose tolerance test but after a normal meal, which means calorie and nutrient intakes may have varied from subject to subject. In addition, study subjects with various medications, such as metformin, sulfonylurea, and DPP-4 I, could be another limitation as well, because these medications might affect serum levels of insulin and glucagon. However, statistical significance was still prominent even after adjusting for all three medications, and this may also strengthen our main findings. Conversely, our data may reflect “everyday” changes in glucagon, insulin, and glucose in actual clinical practice.
In summary, type 2 diabetes patients with higher glucagon-to-insulin ratios, especially in a postprandial state, were more likely to show uncontrolled hyperglycemia. Accordingly, we suggest that different treatment approaches may be needed for subjects with relatively lower BMI, less insulin resistance, and dysregulated glucagon levels, compared to patients with higher BMI and more insulin resistance. Instead of concentrating on improvements in insulin resistance, a strategy to suppress glucagon together with increasing insulin secretion may be another key therapeutic option for treating type 2 diabetic patients in the future. Understanding the diverse pathogenesis of hyperglycemia may help to personalize treatment strategies in type 2 diabetes. Larger studies to investigate the effect of glucagon together with the action of insulin are warranted.
KEY MESSAGE
1. Hyperglucagonemia relative to insulin could contribute to uncontrolled hyperglycemia in type 2 diabetes patients.
2. A strategy to suppress glucagon together with increasing insulin secretion may be another key therapeutic option for treating type 2 diabetic patients with lower body mass index and less insulin resistance.
Notes
No potential conflict of interest relevant to this article was reported.
Acknowledgements
This research was supported by a grant from the Korea Health Technology R&D Project through the Korea Health Industry Development Institute (KHIDI), funded by the Ministry of Health and Welfare, Republic of Korea (grant number: HI14C0336). The authors are grateful to Dr. Lee and Ms. Hong (the Biostatistics Collaboration Units of Yonsei University College of Medicine) for their advice on the statistical analyses.