Phosphodiesterase Inhibitor Improves Renal Tubulointerstitial Hypoxia of the Diabetic Rat Kidney
Article information
Abstract
Background/Aims
Renal hypoxia is involved in the pathogenesis of diabetic nephropathy. Pentoxifyllin (PTX), a nonselective phosphodiesterase inhibitor, is used to attenuate peripheral vascular diseases. To determine whether PTX can improve renal hypoxia, we investigated its effect in the streptozocin (STZ)-induced diabetic kidney.
Methods
PTX (40 mg/kg, PO) was administered to STZ-induced diabetic rats for 8 weeks. To determine tissue hypoxia, we examined hypoxic inducible factor-1α (HIF-1α), heme oxygenase-1 (HO-1), vascular endothelial growth factor (VEGF), and glucose transporter-1 (GLUT-1) levels. We also tested the effect of PTX on HIF-1α in renal tubule cells.
Results
PTX reduced the increased protein creatinine ratio in diabetic rats at 8 weeks. HIF-1α, VEGF, and GLUT-1 mRNA expression increased significantly, and the expression of HO-1 also tended to increase in diabetic rats. PTX significantly decreased mRNA expression of HIF-1α and VEGF at 4 and 8 weeks, and decreased HO-1 and GLUT-1 at 4 weeks. The expression of HIF-1α protein was significantly increased at 4 and 8 weeks in tubules in the diabetic rat kidney. PTX tended to decrease HIF-1α protein expression at 8 weeks. To examine whether PTX had a direct effect on renal tubules, normal rat kidney cells were stimulated with CoCl2 (100 µM), which enhanced HIF-1α mRNA and protein levels under low glucose conditions (5.5 mM). Their expressions were similar even after high glucose (30 mM) treatment. PTX had no effect on HIF-1α expression.
Conclusions
PTX attenuates tubular hypoxia in the diabetic kidney.
INTRODUCTION
Chronic hypoxia is involved in the pathogenesis of chronic renal injury, such as diabetic nephropathy. Hypoxic injury can induce tubulointerstitial fibrosis and atrophy [1,2]. These tubulointerstitial changes are associated with structural and functional deterioration of the renal vasculature [3-5].
Glomerular and tubular hypoxia may be induced by hyperglycemia or dysregulation of the glomerular arterioles in diabetic nephropathy. Intra-renal oxygen tension decreases in the diabetic kidney, especially in the outer medullary region [6,7]. The changes in intrarenal microcirculation are unclear, but oxygen consumption is increased in the diabetic kidney, suggesting its vulnerability to hypoxia [8].
Hypoxia impairs various cellular functions. Hypoxic cells synthesize hypoxia-inducible transcription factors (HIF) and heme oxygenase (HO) to minimize tissue damage. HIF, which is one of the most important hypoxia-related molecules, plays a role in adaptation to a low oxygen supply [9,10]. HIF is produced in response to hypoxia in various renal cell types [11].
Cyclic-3', 5'-phosphdiesterase (PDE) degrades cyclic-3', 5'-adenosine monophosphate and cyclic 3', 5'-guanosine monophosphate affecting their intra- and extra-cellular concentrations [12]. PDE 3 inhibitor improves endothelial function and inflammatory changes in damaged vascular smooth cells stimulated by tumor necrosis factor-α [13,14]. The PDE 4 inhibitor rolipram also reduces expression of adhesion molecules, such as P-selectin and E-selectin, which are critical substances in the binding of white blood cells to endothelial cells [15]. In the clinical field, PDE inhibitors are used to treat peripheral vascular and bronchoconstrictive diseases [16,17].
Despite the fact that hypoxia is involved in the pathogenesis of diabetic nephropathy, restoration of renal tubular hypoxia is difficult. Based on the hypothesis that PDE inhibitors may improve renal tubular hypoxia, we investigated whether pentoxifyllin (PTX), a nonselective PDE inhibitor, may attenuate the hypoxic condition in streptozocin (STZ)-induced type 1 diabetic rats.
METHODS
Animal study and experimental design
Male Sprague-Dawley rats weighing 200-220 g were divided into three groups. Twelve rats in which diabetes mellitus was not induced were used as controls. Diabetes mellitus was induced by intraperitoneal injection of STZ, 65 mg/kg body weight (Sigma-Aldrich, Saint Louis, MO, USA). Diabetic rats (n = 26) were further randomized into a diabetic control group and a treatment group. Diabetes in the rats was confirmed by a > 300 mg/dL tail blood sugar concentration 48 hours after STZ injection. Rats in the PTX group were treated with 40 mg/kg PTX (Sigma-Aldrich). Fourteen of the rats were sacrificed and the remainder were kept for an additional 4 weeks. Six-hour urine was collected using metabolic cages. Then the rats were anesthetized by intraperitoneal injection of sodium pentobarbital (50 mg/kg). The cortex of one kidney in Trizol reagent (Invitrogen, Carlsbad, CA, USA) was stored at -70℃. The other kidney was perfused with normal saline and fixed in 4% paraformaldehyde.
Body and kidney weights were measured upon sacrifice. Blood glucose levels were determined using standard techniques. Urinary protein was measured with a Pierce BCA Protein Assay Kit (Pierce Biotech Inc., Rockford, IL, USA), and then normalized to urine creatinine measured by the Alkaline Picrate method.
Cell culture and experimental design
Immortalized normal rat kidney (NRK) cells were acquired from the American Tissue Culture Collection (Rockville, MD, USA). These cells were cultured in DMEM (GibCo BRL, Carlsbad, CA, USA), supplemented with 10% heat-inactivated fetal bovine serum (FBS), 100 U/mL penicillin, and 100 mg/mL streptomycin at 37℃ in a humidified atmosphere of 95% air and 5% CO2. Then subconfluent cells were cultured in 0.1% FBS for 24 hours before use in experiments. The cells were incubated in 5.5 mM (normal glucose, NG) or 30 mM (high glucose, HG) D-glucose. To induce hypoxia, 100 µM CoCl2 was administered 6 hours prior. Cells were pretreated with PTX 30 minutes before drug treatment. All experimental groups were cultured in triplicate and harvested at 6 and 24 hours for extraction of total RNA and protein.
Real-time reverse transcriptase-polymerase chain reaction (RT-PCR)
Total RNA was extracted from renal cortical tissues or cells with Trizol reagent. Total RNA was reverse transcribed into cDNA using cDNA synthesis (Fermentas, Burlington, Canada) and RNA PCR (Applied Biosystems, Carlsbad, CA, USA) kits in a 20 µL mixture containing 0.5 µg RNA, 50 mM KCl, 50 mM Tris/HCl, 4 mM MgCl2, 1 mM of each dNTP, oligo-(dT) primers, 20 units of RNAse inhibitor, and 50 units of MuLV reverse transcriptase. The reaction mixture was incubated for 10 minutes at 37℃, 60 minutes at 42℃, and then heated at 90℃ for 5 minutes in a thermocycler (GeneAmp PCR system 2700, Applied Biosystems). RT-PCR was performed using standard three-step cycling conditions with SYBR Green Mastermix. Primers were designed from the respective gene sequences using the Primer 3 software. The nucleotide sequences of each primer were as follows: HIF-1α (sense 5' ACAGCACATTCACAGCTCCCCA, anti-sense 5' TGTGGCTACCATGTACTGCTGGC3'), HO-1 (sense GTGCCCTTGGTAGTCGGCGT, anti-sense CCTCAAAAGACAGCCCTACTTGGTT), vascular endothelial growth factor (VEGF; sense AGCCTTGTTCAGAGCGGAGAA, anti-sense TAACTCAAGCTGCCTCGCCTT), glucose transporter-1 (GLUT-1; sense CAAACTGACAGATCTCGGGC, anti-sense TTCTGGAGCCATCAAAGTCC), glyceraldehyde-3-phosphate dehydrogenase (GAPDH; sense TGCACCACCAACTGCTTAGC, anti-sense 5'-GGCATGGACTGTGGTCATGAG-3'). The expression levels of all genes were normalized to that of the housekeeping gene GAPDH.
Immunohistochemical staining for HIF-1α and HO-1
For immunohistochemical staining, renal tissue was immediately fixed in 10% neutral buffered formalin, cast in paraffin, and then sliced into 4 µm thick sections. After removal of paraffin in xylene and dehydration in a graded alcohol series, slides were immersed in distilled water. Kidney sections were transferred to a 10 mM citrate buffer solution for antigen retrieval at a pH of 6.0 and then incubated at a sub-boiling temperature for 15 minutes. After washing with water, 3.0% H2O2 was applied for 15 minutes to block endogenous peroxidase. To inhibit nonspecific staining, slides were incubated at room temperature for 1 hour with normal goat serum. The primary antibodies mouse monoclonal antihuman HIF-1α (Chemicon, Billerica, MA, USA, 1:500) and rabbit polyclonal anti-rat HO (Abcam, Cambridge, MA, USA, 1:1,000) were added to the slides followed by overnight incubation at 4℃. Negative control sections were stained under identical conditions by substituting the primary antibody with equivalent concentrations of normal rabbit IgG.
Expression was evaluated by semi-quantitatively grading staining within tubules. In this method, each score reflects changes in the extent rather than in the intensity of staining, and depends on the percentage of the grid field that stains positive. Four scores were awarded: 0, absent or < 25% positive staining; 1, 25-50% positive staining; 2, 50-75%; and 3, > 75% positive staining. The average score of each sample was calculated. Each slide was scored by an observer blinded to the experimental details.
Western blotting
Cells were lysed in buffer (150 mM NaCl, 50 mM Tris-HCl, pH 8.0, 1% Triton X-100, 1 mM phenylmethylsulfonylfluoride), and total protein concentrations were quantified using the advanced protein assay reagent (Bio-Rad, Hercules, CA, USA) in a spectrophotometer. An aliquot of 40 µg protein was electrophoresed on 6% or 10% SDS-polyacrylamide gels under denaturing conditions. Then proteins were transferred to polyvinylidene difluoride membranes (Immobilon-P, Millipore, Billerica, MA, USA) for 120 minutes at 250 mA. After blocking in blocking solution (PBS, 0.15% Tween-20, 5% non-fat milk) for 1 hour at room temperature, membranes were hybridized overnight at 4℃ using mouse monoclonal anti-human HIF-1α (Chemicon, 1:500), rabbit polyclonal ant-rat HO-1 (Abcam, 1:1,000), and antirat β-actin (Abcam, 1:3,000). Then the membranes were washed four times with PBS-Tween-20, and incubated for 30 minutes at room temperature with horseradish peroxidase-conjugated secondary antibody, diluted to 1:3,000. Specific signals were detected using the enhanced chemiluminenscence method (Amersham, Buckinghamshire, UK). Equal protein loading was confirmed by β-actin.
Statistical analysis
We used a nonparametric analysis because most variables were not normally distributed, even after logarithmic transformation. The t test was used to compare groups, and a value of p < 0.05 was defined as statistically significant. All statistical analyses were performed using SPSS version 10.0 (SPSS Inc., Chicago, IL, USA). Data are expressed as mean ± standard derviation.
RESULTS
Baseline characteristics of diabetic rats
Blood glucose concentration and kidney weight were significantly higher in STZ-induced diabetic rats than in control rats at 4 and 8 weeks. The increased blood glucose concentration and kidney weight were unaffected by PTX treatment.
The protein creatinine ratio was significantly higher in diabetic rats than in control rats at 4 and 8 weeks. This increased ratio decreased in diabetic rats after PTX treatment at 8 weeks (13.9 ± 4.61 vs. 9.88 ± 1.67 mg/mgCr; p < 0.05) (Table 1).
Hypoxia-related factor mRNA levels
HIF-1α, VEGF, and GLUT-1 mRNA levels were significantly higher in diabetic rats; that of HO-1 tended to increase in diabetic rats. PTX significantly decreased both HIF-1α and VEGF mRNA expression at 4 and 8 weeks, and decreased that of HO-1 and GLUT-1 at 4 weeks (Fig. 1).
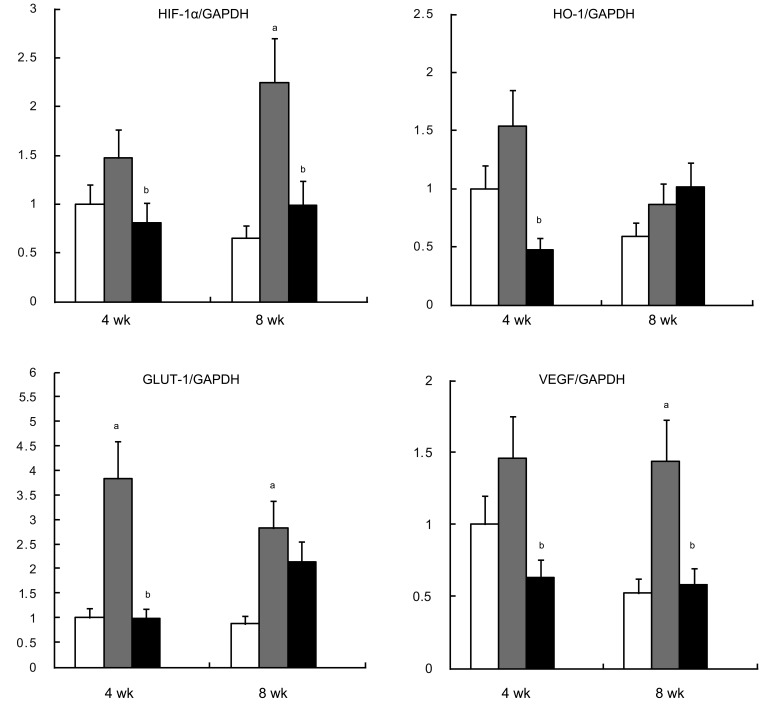
mRNA expression of HIF-1α (A), HO-1 (B), GLUT-1 (C), and VEGF (D). Values are presented mean ± SD. HIF-1α, hypoxic inducible factor-1α; GAPDH, glyceraldehyde-3-phosphate dehydrogenase; HO-1, heme oxygenase-1; GLUT-1, glucose transporter-1; VEGF, vascular endothelial growth factor. ap < 0.05, diabetic rats (gray bar) vs. control rats (white bar); bp < 0.05, diabetic rats with pentoxifyllin (black bar) vs. diabetic rats.
Immunohistochemical staining for HIF-1α and HO
HIF-1α synthesis in the diabetic rat kidney increased at both 4 and 8 weeks. At 8 weeks, its expression was consistently higher than that in control rats. HO-1 expression was not different compared to control rats. These molecules were expressed mainly in the tubules, not in the glomeruli. PTX tended to decrease HIF-1α protein expression by 36% at 8 weeks (Figs. 2 and 3).
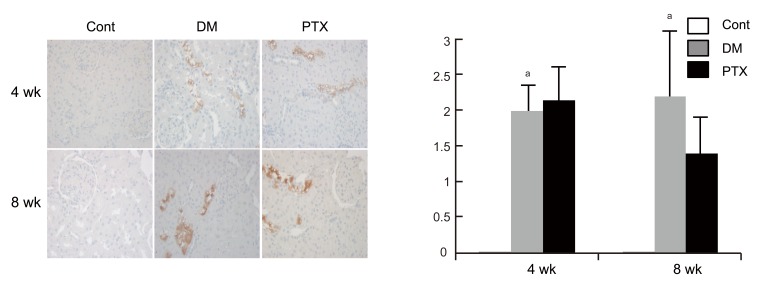
Hypoxic inducible factor-1α (HIF-1α) immunohistochemistry in experimental animals. Representative HIF-1α immunostaining findings (A) and scores (B) in control (Cont), diabetic rats (DM), and DM with pentoxifyllin (PTX). HIF-1α are strongly stained in the distal tubule of the DM and PTX groups, whereas no staining in the control group. Values are presented mean ± SD. ap < 0.05, diabetic rats (gray bar) vs. control rats (white bar).
Effect of PTX on CoCl2-stimulated NRK cells
To investigate whether PTX directly affected renal tubules, NRK cells were stimulated with CoCl2 (100 µM), which induced HIF-1α mRNA production under NG conditions. HIF-1α mRNA was also increased under HG conditions. Production was similar even after HG treatment. HG and CoCl2 showed no synergistic effect on HIF-1α. HIF-1α protein showed a similar pattern. HO-1 mRNA production tended to increase in response to CoCl2, and protein expression was similar to mRNA expression (Fig. 4).
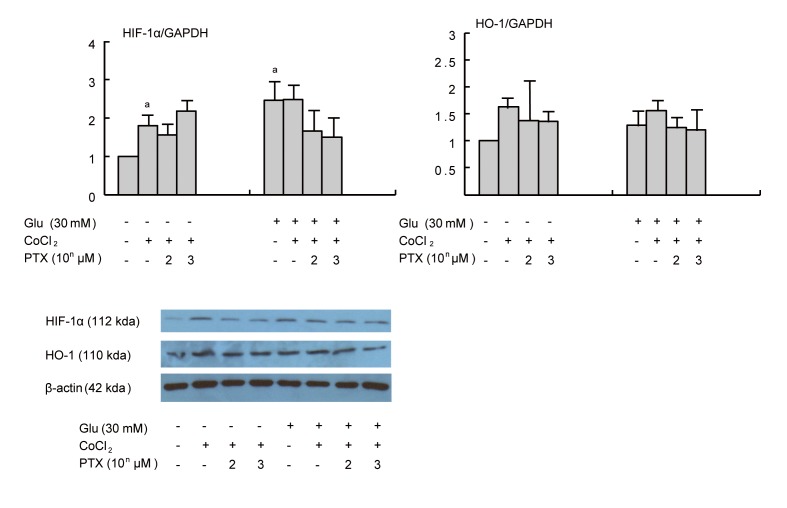
Effect of pentoxifyllin (PTX) on hypoxic inducible factor-1α (HIF-1α) mRNA (A), and heme oxygenase-1 (HO-1) mRNA (B) and protein (C) expression in cultured normal rat kidney cells (NRK) cells. CoCl2 stimulated HIF-1α in normal glucose (NG). There was no synergistic effect of HG and CoCl2 on HIF-1α. HIF-1α exhibited a similar pattern. Values are presented mean ± SD. ap < 0.05, vs. NG.
DISCUSSION
PTX reduced HIF-1α and VEGF expression, which are upregulated in diabetic rats, but had no direct effect on HIF-1α in NRK cells stimulated with CoCl2 under both NG and HG conditions. These results suggest that PTX attenuates renal hypoxia in the diabetic kidney.
The effect of hyperglycemia on HIF expression is unclear, and depends on cell type. Hyperglycemia inhibits HIF expression, affecting cell survival [18,19]. VEGF is also suppressed by hypoxic stimuli in diabetic rats [20]. However, hyperglycemia itself stimulates hypoxia-related molecules [8]. In the present study, CoCl2 upregulated HIF-1α expression in renal tubule cells, but did not further affect HIF-1α expression after HG stimulation.
Hypoxia plays an important role in the pathogenesis of diabetic nephropathy due to the relative hypoxia induced by peritubular flow disturbance in hyperfiltration induced by hyperglycemia [21-23]. In addition, peritubular capillary damage reduces renal blood flow, subsequently increasing the intrarenal oxygen requirement [7,24]. There have been attempts to improve renal hypoxia using erythropoietin, prolyl hydroxylase inhibitor, and VEGF [25-28]; however, the efficacies of these compounds are not clear. A recent trial directly increased intrarenal HIF and HO expression to reduce renal tubular damage [29].
The renoprotective effect of PTX in diabetic nephropathy is unclear. Although a metaanalysis showed that PDE inhibitor reduces proteinuria in patients with diabetic nephropathy, PTX has not been used in patients with diabetic nephropathy [30]. In animal studies, PDE inhibitors stimulated lipolysis and free fatty acid synthesis, and improved insulin resistance and endothelial dysfunction [14,31,32]. It also affects peripheral and renal blood flow [33,34], suggesting the possibility of attenuating renal tubular blood flow. In the present study, PTX did not influence HIF-1α expression in NRK cells stimulated with CoCl2 and HG. The mechanism underlying the beneficial effect of PTX in the STZ-induced diabetic kidney seems to be related to improving renal blood flow by attenuating peritubular endothelial dysfunction, rather than having a direct effect. The mechanism underlying the beneficial effect of PTX on renal hypoxia could be confirmed by measuring renal tubular blood flow.
In conclusion, PTX, a peripheral vasodilator, reduced renal hypoxia in diabetic rats. In the future, direct measurements of blood flow will further clarify the effect of PTX.
Acknowledgements
This work was supported by a 2009 Korean Association of Internal Medicine Research Grant.
Notes
No potential conflict of interest relevant to this article was reported.