Advanced heart failure: a contemporary approach
Article information
Abstract
Advanced heart failure (HF) is defined as the persistence of severe symptoms despite the use of optimized medical, surgical, and device therapies. These patients require timely advanced treatments, such as heart transplantation or long-term mechanical circulatory support (MCS). Inotropic agents are often used to reduce congestion and increase cardiac output, while renal replacement therapy may be beneficial if necessary. Cardiac resynchronization therapy has clear benefits in patients with HF with reduced ejection fraction, particularly with left bundle branch block (QRS duration > 130 ms). The role of implantable cardioverter-defibrillators in advanced HF patients requires further investigation considering the introduction of novel HF medications. In selected patients with significant secondary mitral regurgitation, transcatheter edge-to-edge repair can help delay heart transplantation or long-term MCS. In later stages, the appropriateness of heart transplantation should be evaluated, and the use of short- or long-term MCS may be considered. A multidisciplinary HF management program is crucial for patients with advanced HF. Recent treatment advances, including drugs, devices, and MCS, have broadened the options available to patients with advanced HF and this trend is expected to continue.
INTRODUCTION
Heart failure (HF) is a complex progressive clinical disease that constitutes a significant burden due to its high mortality and morbidity rates, and consequent challenge on healthcare systems worldwide [1,2]. In Korea, more than 1 million adults have HF, with 300,000 new cases diagnosed annually [3,4]. The management of HF has changed dramatically over the past few decades; it incurs substantial healthcare costs and is currently the foremost cause of hospitalization, with about 100,000 patients being admitted annually in Korea [3].
Medical therapy is a fundamental cornerstone of HF treatment and interventional therapies including devices, catheter-based procedures, or surgery are essential for selected patients with HF to ensure survival, preserve cardiac function, and improve quality of life and prognosis. Despite the implementation of evidence-based therapies that enhance outcomes for patients with chronic HF, the disease can still progress over time. The increasing prevalence of advanced HF can be attributed to the growing population of patients diagnosed with HF, along with the elderly and those with multiple comorbidities. However, young patients may also present with advanced HF.
Despite improvement in the management of advanced HF, the prognosis for these patients remains unfavorable, with an estimated 1-year mortality rate between 25% and 75% [5,6]. The estimated prevalence of advanced HF ranges between 5% and 25% in the HF population [7,8]. It is imperative to establish a precise comprehensive definition of advanced HF to enable accurate identification and timely utilization of advanced treatments, including heart transplantation and long-term mechanical circulatory support (MCS).
Here, we present a comprehensive review of advanced HF, providing a clear definition of the condition and an in-depth discussion of its contemporary management.
DEFINITION AND CLASSIFICATION OF ADVANCED HEART FAILURE
Advanced HF is commonly considered the presence of progressive or persistent severe symptoms and signs of HF despite optimized medical, surgical, and device therapy. The 2018 European Society of Cardiology guideline defines advanced HF (Table 1) [9]. Although a severely reduced left ventricular ejection fraction (LVEF) is common, it is not mandatory for a diagnosis of advanced HF as it may also occur in patients with HF with a preserved or mildly reduced ejection fraction [10]. Apart from the reported criteria for advanced HF, extracardiac manifestations of HF (e.g., cardiac cachexia or liver or kidney dysfunction) or type II pulmonary hypertension may be present but are independent of its definition. The United States American College of Cardiology/American Heart Association (ACC/AHA) definition categorizes HF as stages A through D; “stage D” and “advanced” are used interchangeably in the relevant documents, including “end-stage” and “refractory” HF [11,12]. Clinical signs and symptoms of advanced HF warrant referral to a specialist in HF. These additional indicators include intolerance to renin-angiotensin-aldosterone system inhibitors or beta-blockers, diuretic resistance, progressive decline in renal function, persistent hyponatremia, and frequent implantable cardioverter-defibrillator (ICD) shocks, etc [11].
Advanced HF can present acutely in patients who experience events such as cardiogenic shock due to acute myocardial infarction or fulminant myocarditis. These patients differ from those with chronic HF who progress gradually to stage D. However, their management is equally challenging due to the paucity of data that can guide clinical decision-making in such cases. Therefore, the need for criteria to differentiate between different patterns of clinical progression in advanced HF emerged, leading to the development of the Interagency Registry for Mechanically Assisted Circulatory Support (INTERMACS) profiles (Table 2) [13,14]. This profiling system delineates clinical characteristics that indicate the need for advanced therapies and categorizes patients with advanced HF who may benefit from durable MCS devices. It can also predict the prognosis of patients undergoing urgent heart transplantation [15] or implantation of a left ventricular assist device (LVAD) [16], and evaluate the risk for ambulatory patients with advanced HF [17]. Use of the INTERMACS classification system is crucial for selecting patients who are suitable for referral to an advanced HF center to facilitate the appropriate application of advanced treatments, such as heart transplantation or MCS.
CONTEMPORARY THERAPEUTIC APPROACHES
Patients with advanced HF frequently need pharmacological therapy or a temporary MCS while awaiting a long-term MCS or heart transplantation. For HF with reduced ejection fraction (HFrEF), the treatment involves the initiation and maintenance of guideline-directed medical therapy (GDMT), including four-pillar medications such as angiotensin-converting enzyme inhibitors/angiotensin receptor antagonists or angiotensin receptor-neprilysin inhibitors (ARNIs), mineralocorticoid receptor antagonists, beta-blockers, and sodium-glucose cotransporter 2 (SGLT2) inhibitors. If patients have worsening HF symptoms or progressive decline despite maintenance GDMT [18], the treatment strategies for advanced HF in the following sections should be considered.
MEDICAL THERAPIES
Inotropes
In acute decompensated HF, inotropic agents may have a positive effect on hemodynamic parameters by reducing congestion, increasing cardiac output, and improving peripheral perfusion. The use of traditional inotropic agents may also lead to myocardial ischemia or tachyarrhythmias and exacerbate the clinical course of the disease [19,20]. Despite the potential risks associated with inotropic agents, they can be administered to a subgroup of HF patients who are unresponsive to other therapies and are experiencing end-organ hypoperfusion [10,11]. In patients with cardiogenic shock, norepinephrine is preferred over epinephrine [21,22] or dopamine [23], while milrinone and dobutamine have comparable outcomes as inotropes based on recent studies [24,25]. The calcium sensitizer levosimendan can be considered for patients with acute decompensated HF on beta-blockers because its mechanism of action differs from that of dobutamine [26]. Meta-analyses have shown that levosimendan in advanced HF patients improves survival and lowers hospital readmission rates [27,28].
Note that the decision to use continuous inotrope infusions in the home setting or a transitional therapy to facilitate a patient’s candidacy for advanced therapies, such as MCS, should be based on an individualized approach after a careful discussion with the patient and his or her family [29]. The potential risks and benefits of such a treatment option should be weighed carefully and discussed with the patient and their caregivers. In general, this treatment should be reserved for patients with advanced HF who have exhausted all other treatment options and have a limited life expectancy. Close monitoring and follow-up by a healthcare provider are essential to ensure the safe effective use of inotropes in these patients.
Renal replacement therapy
Kidney dysfunction is a common comorbidity in patients with HF, and the clinical course of advanced HF is often characterized by diuretic resistance [30]. If volume overload persists despite the use of stable diuretic doses, the loop diuretic dose can be doubled initially, followed by the concomitant administration of other types of diuretics, such as thiazides or metolazone [31]. In patients with acute decompensated HF, the Diuretic Optimization Strategies Evaluation (DOSE) trial revealed that the continuous infusion of loop diuretics and intermittent bolus treatment had similar clinical outcomes [32]. In a small retrospective study that investigated drug-refractory advanced HF, intermittent renal replacement therapy was associated with a decreased risk of death or LVAD implantation compared to continuous inotrope infusion [33]. Particularly, patients in cardiogenic shock are at risk for hemodynamic instability due to fluid shifts that can occur during intermittent hemodialysis. Continuous renal replacement therapy, which gradually removes fluid and toxins through the application of a veno–venous driving force using an external pump, is more commonly used for renal replacement therapy in this population [14]. While renal replacement therapy can be considered for patients with diuretic resistance, there is still little conclusive data on its outcomes, so it also requires individualized treatment [10].
DEVICE THERAPIES
Cardiac resynchronization therapy with novel techniques
While cardiac resynchronization therapy (CRT) is clearly helpful and is recommended for patients with HFrEF, there are limited data on its efficacy in advanced HF patients (e.g., New York Heart Association [NYHA] class IV), accounting for less than 10% of CRT clinical studies [34]. In the randomized controlled Comparison of Medical Therapy, Pacing, and Defibrillation in HF (COMPANION) trial, which included patients with NYHA class III to IV and a QRS of 120 ms or greater, both CRT with a pacemaker (CRT-P) or defibrillator (CRT-D) were found to reduce the primary endpoints of death or hospitalization compared to a group that receive only GDMT [35]. In the subgroup with ambulatory NYHA class IV, CRT-P and CRT-D therapy delayed the times to death and hospitalization, and tended to improve survival. The time to sudden death was significantly reduced in the CRT-D group, suggesting that CRT improves outcomes in some advanced HF patients, but the benefit is less certain in inotrope-dependent patients [36]. Studies have observed that patients with substantial LV dilation and less dyssynchrony have lower chances of reverse remodeling and survival following CRT [37]. Although there is currently insufficient evidence to determine whether CRT implantation is appropriate for advanced HF patients, any decision should be individualized and based on the overall goal.
Recently, left bundle branch pacing (LBBP) has been introduced as an alternative to conventional biventricular pacing (BVP) for CRT. LBBP paces the left bundle branch directly, which is reported to have a higher success rate and broader indications than BVP mode [38,39]. Left bundle branch area pacing (LBBAP) techniques have also been developed, including both LV septal pacing and LBBP; these are similar to physiological LV activation [39–41]. However, for patients with severe electrical dyssynchrony in advanced HF, LBBAP alone may not always provide optimal electrical synchronization. To address this, LBBAP in combination with BVP (sequential LV pacing) has been explored as a potential solution to improve synchronization and clinical outcomes. Recently, Jastrzebski et al. [42] investigated LBBAP-optimized CRT (LOT-CRT) in patients in whom CRT was indicated or were non-responders to BVP alone. The study included patients with severe dyssynchrony and a wide QRS (mean 181 ms). The results showed that LOT-CRT resulted in greater QRS narrowing compared to either BVP or LBBAP alone, indicating better electrical synchrony in this specific population. In addition, LOT-CRT showed more reverse-remodeling in LV and improvement of the NYHA class compared to either BVP or LBBAP alone. However, further research is needed to understand its efficacy fully.
Implantable cardioverter-defibrillator
The ICD can abort sudden cardiac death (SCD); therefore, it is primarily indicated for the primary prevention of SCD in advanced HF. However, it does not improve symptoms in this population, so the higher the risk for SCD, the greater the expected benefit from ICD in each patient. In 2002, the Multicenter Automatic Defibrillator Implantation Trial II (MADIT-II) reported that patients with a previous myocardial infarction and LVEF ≤ 30% derived survival benefits from ICD [43]. However, given that more than 70% of the patients enrolled in this study were NYHA class I–II, the results are not directly applicable to advanced HF. In the Sudden Cardiac Death in HF Trial (SCD-HeFT), the use of an ICD significantly reduced mortality compared to amiodarone therapy [44]. However, this effect was not observed in patients with advanced symptoms. The Defibrillator Implantation in Patients with Nonischemic Systolic HF (DANISH) trial published in 2016 included patients with nonischemic cardiomyopathy and LVEF ≤ 35% with or without an ICD; there were no significant differences in the long-term mortality rates between the two groups [45]. Collectively, these studies indicate that the role of ICDs in advanced HF requires further investigation, given that the introduction of novel HF medications such as ARNIs and SGLT2 inhibitors has enhanced overall outcomes since the time of these studies.
Transcatheter edge-to-edge mitral valve repair
Typically, LV dysfunction negatively affects mitral valve closure, leading to asymmetric closure, and severe LV dilation promotes symmetrical tethering of both valve leaflets resulting mitral regurgitation (MR) [46]. Conventionally, MR reflects the severity of the underlying LV dysfunction [47], and functional MR with a structurally normal valve is classified as secondary MR [48]. However, there is growing evidence that asymmetric mitral leaflet dysfunction can occur in patients with global LV dysfunction because it does not necessarily affect the chamber in a homogenous manner [46]. Patients with disproportionate MR who have severe MR that cannot be explained by the severity of LV dilation should be differentiated from those with proportionate MR because they respond poorly to medical therapies [49]. For such patients, a transcatheter edge-to-edge mitral valve repair (TEER) for MR is a therapeutic option if they have persistent symptoms after GDMT [50]. In the Cardiovascular Outcomes Assessment of the MitraClip Percutaneous Therapy for Heart Failure Patients with Functional Mitral Regurgitation (COAPT) study, most enrolled patients had disproportionate MR, with only 10% of the patients with proportionate MR having an LV end-diastolic volume index > 96 mL/m2 and effective regurgitant orifice area ≤ 30 mm2. In a post hoc analysis of this study, TEER was accompanied by a significant risk reduction of all-cause mortality and HF hospitalization [46]. However, MR proportionality is a conceptual framework, so selecting patients based on this proportionate–disproportionate hypothesis alone is still questionable. According to recent European registry data, patients with advanced HF awaiting heart transplantation, most of whom had proportionate MR, underwent TEER as a bridge strategy and two-thirds of them remained free from a composite event of death, urgent heart transplant or LVAD implantation, and HF hospitalization [51]. Therefore, robust evidence for TEER in advanced HF is still needed, and the physician’s judgment currently remains an important part of the decision in most cases, along with echocardiographic parameters for MR.
Mechanical circulatory support
MCS can be classified into short- and long-term devices for managing patients with advanced HF or cardiogenic shock [14]. There is currently insufficient high-quality evidence to establish the optimal use of MCS devices as a therapeutic intervention for patients with circulatory failure. Existing supportive data are primarily based on small randomized trials with hemodynamic endpoints, or observational studies that have demonstrated improved survival rates in selected patients compared to historical controls and clinical experience [52–54]. For patients with cardiogenic shock, the INTERMACS registry showed that the 1-month mortality for INTERMACS profiles 1 and 2 reached 38% [55], and the early mortality after MCS implantation remained very high [56,57].
Short-term mechanical circulatory support
Temporary (short-term) MCS may be performed for a variety of purposes in patients with circulatory failure who present with hemodynamic instability despite full medical therapy. It can be used for a few days to a few weeks depending on the individual’s clinical status. It is performed as a bridge to recovery (BTR) to wait for cardiac function to recover; when recovery is unlikely, it can be performed as a bridge to bridge (BTB) or bridge to decision (BTD) strategy for either long-term MCS or heart transplantation in patients with INTERMACS profiles 1 or 2 [58], bridge to transplant (BTT) when heart transplantation is urgently required, or as a bridge to candidacy (BTC) strategy when heart transplantation is not immediately possible due to contraindications in other organs, such as cerebral dysfunction [10] (Fig. 1).
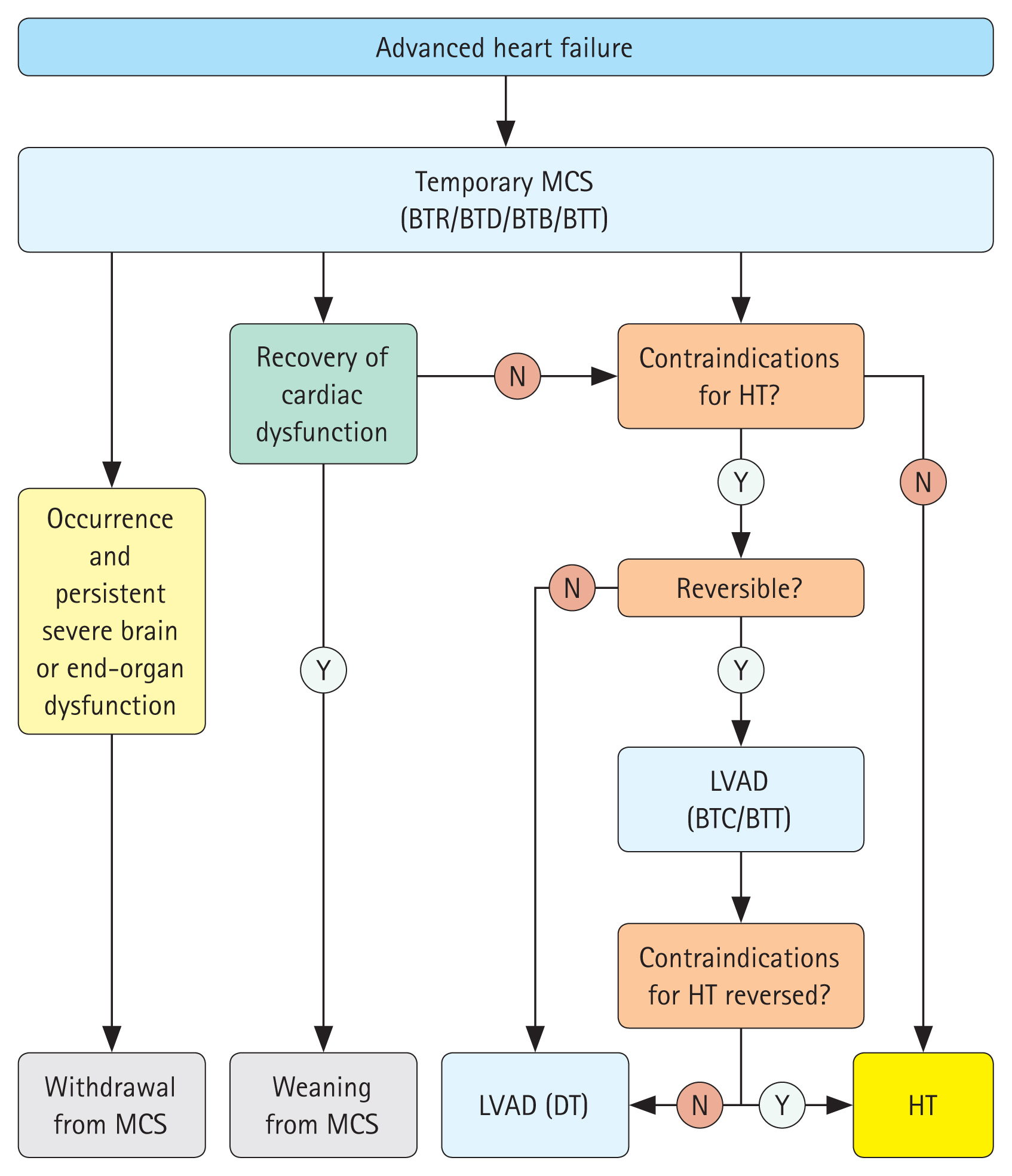
Algorithm of the treatment of patients with advanced heart failure. BTB, bridge to bridge; BTC, bridge to candidacy; BTD, bridge to decision; BTR, bridge to recovery; BTT, bridge to transplantation; DT, destination therapy; HT, heart transplantation; LVAD, left ventricular assist device; MCS, mechanical circulatory support. Modified from McDonagh, et al. Eur Heart J 2021;42:3599-3726 [10] with original copyright holder's permission.
Temporary percutaneous MCS devices include the TandemHeart and Impella systems, etc. [14]. However, these devices are not currently available in Korea. Although the intra-aortic balloon pump (IABP) is still available, its use declined after the IABP-SHOCK II trial, which enrolled patients with myocardial infarction-related cardiogenic shock, showed that it did not reduce mortality compared to those without IABP support [59–61]. The most commonly used temporary MCS in Korea is extracorporeal membrane oxygenation (ECMO) [62]. Veno–arterial ECMO supports both the cardiovascular and respiratory systems and is frequently used to treat circulatory failure. Due to the peripheral access commonly used, its potential complications include ischemic limb damage, infection, thromboembolism, stroke, bleeding, and hemolysis [63]. Peripheral insertion often results in an increased LV afterload, which can cause insufficient LV unloading. Several techniques can be used to address this issue, including ECMO in conjunction with IABP, atrial septostomy, Impella support, and other venting techniques to achieve more suitable ventricular decompression [14,64].
Although the peripheral access is commonly used method, central ECMO can use various arterial cannulation sites. Central veno-arterial ECMO can be performed via the innominate, axillary, or subclavian arteries, or even the ascending aorta [65–67]. Performing ECMO through a central approach has several advantages over peripheral ECMO. For example, a venous cannula can be introduced directly in the left atrium to decompress the LV optimally; it can be useful when there is a high risk for distal limb ischemia due to poor peripheral vascularity; and it can promote patient mobility and theoretically lower the risk for cerebral hypoperfusion and aortic root thrombosis [65,68]. While more evidence is needed, central ECMO is an option when considering MCS for BTC or BTT depending on the patient’s condition.
Long-term (durable) mechanical circulatory support
In specific patients, long-term (durable) MCS is recommended when the maximally optimized medical therapy is insufficient or when short-term MCS has not resulted in cardiac improvement, with the goal of improving survival and quality of life. It may also be used to maintain the patient’s life until heart transplantation, while waiting for contraindications to heart transplantation to resolve, or as destination therapy (DT) [10]. The most established preferred therapy for long-term MCS is durable LVAD. Recent trials, including MOMENTUM 3, have shown that the survival benefit of durable LVAD support in advanced HF patients has progressively increased, with a 2-year survival rate of around 80% with newer generation centrifugal-flow type LVADs, which is similar to the early survival after heart transplantation [69]. Moreover, the 2020 INTERMACS reported improved mean survival of more than 4 years for patients receiving LVADs as DT and more than 5 years for patients receiving LVADs as BTT [70]. While durable LVAD support has markedly improved functional capacity and quality of life in various trials [69,71,72], patients are still constrained by their reliance on a battery connected through a percutaneous lead, which limit such benefits.
Durable LVAD should be considered in patients with persistent severe symptoms despite maximal medical and device therapies [10,70]. The U.S. Food and Drug Administration approved long-term MCS as a BTT in 1998 [73], and durable LVADs were first introduced in Korea in 2018 and are increasingly implanted [74]. Although the number of heart transplantations has increased steadily since the early 2000s [3], the demand for heart transplantation markedly exceeds the available donors, with 770 patients currently on the waiting list for a heart transplant as of February 2021. Consequently, the use of LVADs has emerged as a promising treatment option for advanced HF in countries where there is a severe organ donor shortage [75].
Figure 1 shows the decision algorithm for the application of MCS in patients with advanced HF and the subsequent switch to LVAD and HT. Durable LVAD can be implemented in some cases, as either BTT or DT, in patients with INTERMACS profiles 1 to 4, avoiding temporary MCS. Even in patients with INTERMACS profiles 5 and 6 with high-risk characteristics, advanced HF therapies (long-term MCS or heart transplantation) may be considered [10]. However, the outcomes of LVAD in patients with an INTERMACS profile 1 are worse, and patients with profiles 5 to 7 who are ambulatory may not benefit from MCS based on their symptom burden [76]. Absolute contraindications to LVAD include irreversible neurological, renal, or hepatic dysfunction, medical nonadherence, and severe psychosocial limitations, while relative contraindications include age over 80 years for DT, systemic active infection, extended mechanical ventilation duration, impaired cognitive function, severe peripheral arterial disease, untreated malignancy, and lack of social support [11].
HEART TRANSPLANTATION
Heart transplantation is still the gold standard and ultimate treatment option for advanced HF if not contraindicated. The mortality and morbidity benefits of heart transplantation for selected patients with advanced HF are based on observational cohort studies. According to the International Society for Heart and Lung Transplantation [77] and United Network of Organ Sharing [78], adult heart transplant recipients now have a median survival exceeding 12 years. The improvement in the management of patients before and after heart transplantation has led to an increase in the number of eligible transplant candidates and the proportion of patients experiencing rejection in the first year after transplantation has decreased to less than 15% [77]. Nevertheless, allograft injury after heart transplantation is often difficult to recognize or is confused due to its complex symptoms and signs. Immediately after transplant, effects of donor factors (including size mismatch), ischemic time of the surgery, surgical technique, and early rejection should be suspected [79,80]. Rejection should be suspected when there is hemodynamic instability, sudden arrhythmias, or ventricular dysfunction, and additional tests are required in these situations. Newer techniques such as cardiac magnetic resonance imaging, index of microcirculatory resistance, and donor-derived cell-free DNA may be helpful for surveillance of allograft rejection [79,81].
Apart from rejection or primary graft dysfunction, several challenges still follow heart transplantation related to the efficacy or safety of immunosuppression, such as infection, late graft dysfunction, cardiac allograft vasculopathy, and malignancy [10,82,83]. Because the clinical course differs completely after transplantation, appropriate patient selection is crucial. Table 3 summarizes the indications [84] and contraindications [85]. Careful evaluation of patients is necessary to determine if they have been optimally treated with GDMT; have comorbidities, family, and social support; and have established care goals [11]. The decision-making process for heart transplantation or LVAD is complex and individualized for each patient, considering fluctuating medical conditions. As shown in Figure 1, it is important to plan and reassess treatment for advanced HF therapy before taking the next step to heart transplantation.
After the first heart transplantation in Korea in 1992, the number performed annually increased to more than 50 cases between 2000 and 2007, reaching 194 cases in 2019 (Fig. 2). However, the traditional cultural view of reluctance to donate organ has hindered widespread transplantation in Asia, so the number of transplants is growing slowly, unlike the global trend [62]. A substantial population with advanced HF awaiting heart transplantation remains. In this context, there is potential to improve the long-term outcomes of heart transplantation with sophisticated immunosuppressive therapies and watchful surveillance.
MULTIDISCIPLINARY MANAGEMENT
Multiple trials have supported multidisciplinary team-based HF management programs and current guidelines recommend enrolling patients with advanced HF in these programs [11]. A multidisciplinary team typically includes HF cardiologists, cardiothoracic surgeons, nursing staff, pharmacists, physical therapists, nutritionists, and social workers (Fig. 3) [86]. Collaborative management of each patient is essential, because individuals with advanced HF often present with multiple comorbidities and can encounter various problems at each step. For example, frailty is common in elderly patients with HF and a multidisciplinary assessment of frailty may offer additional prognostic information, even in those undergoing LVAD implantation [87,88]. Cardiac rehabilitation in elderly patients is safe and has been shown to improve quality of life [89]. Cardiac rehabilitation should not be overlooked in patients who have received LVAD implantation or heart transplant. Indeed, the multidisciplinary care of these patients with advanced HF should be intensified.
PALLIATIVE AND END-OF-LIFE CARE
Although the treatment of advanced HF has significantly progressed, patients generally experience functional decline and ultimately lead to death. Despite improved survival rates due to advanced therapies, advanced HF progresses to an end-stage and standard treatment options are no longer effective. Advanced treatment for this population may sometimes be detrimental in elderly patients, those with comorbidities (e.g., neurological disability, cardiac cachexia, or sarcopenia), or those with a limited life expectancy due to untreated cancer [90]. For such people, palliative and end-of-life care to enhance quality of life is critical. As in other end-stage diseases, transition to end-of-life care should be considered when quality of life is more important than quantity of life in patients with end-stage HF [91]. To address the underlying comorbidities and coordinate care for advanced HF, the intervention of a specialist is necessary because individualized care, rather than standardized therapy, is often required. Guidelines also recommend offering palliative care to all patients with HF who have contraindications to MCS or heart transplantation [10].
CONCLUSIONS
With advances in medical and device therapy, the natural course of HF has been altered by delaying disease progression and improving survival rates, and this has resulted in an increasing number of patients with advanced HF. Comprehensive evaluation of each patient is essential, followed by goal-oriented team-based care that includes timely advanced HF therapies, such as LVAD and heart transplantation. Recent advances in treatments including drugs, devices, LVADs, and stem cell and gene therapy have increased the number of treatment options for patients with advanced HF. With the increasing number of patients with advanced HF, healthcare providers must implement comprehensive evaluation and goal-oriented team-based care to manage this population effectively.
Notes
CRedit authorship contributions
Kyeong-Hyeon Chun: conceptualization, writing - original draft; Seok-Min Kang: conceptualization, writing - review & editing, supervision
Conflicts of interest
The authors disclose no conflicts.
Funding
None