Obesity-related heart failure with preserved ejection fraction: diagnostic and therapeutic challenges
Article information
Abstract
The prevalence of heart failure with preserved ejection fraction (HFpEF) has increased in recent decades. In particular, obesity-related HFpEF is a distinct and frequently encountered phenotype; however, its diagnosis is complex. Furthermore, the management of obesity-related HFpEF has not been established despite the introduction of promising drugs. This review summarizes the diagnostic challenges, pathophysiology, and therapeutic options for obesity-related HFpEF.
INTRODUCTION
The prevalence of heart failure is increasing rapidly in Korea and worldwide as a result of an aging society and an increase in cardiometabolic comorbidities [1,2]. Approximately 1.2 million Korean adults have developed heart failure as of 2018, and its prevalence has increased nearly three-fold from 2002 to 2018 [2]. Heart failure is categorized into three subtypes based on the left ventricular ejection fraction (LVEF): heart failure with reduced ejection fraction (HFrEF; LVEF ≤ 40%), heart failure with mildly reduced ejection fraction (40 < LVEF < 50%), and heart failure with preserved ejection fraction (HFpEF; LVEF ≥ 50%). Although categorizing heart failure based on the LVEF is arbitrary (considering the continuous nature of LVEF), the pathophysiology and treatment response of HFrEF and HFpEF are different.
HFpEF is increasing in incidence and emerging as a major health problem [3,4]. A Mayo Clinic study reported an increase in the prevalence of HFpEF over 15 years (from 38% to 54%). More recent data from the Framingham study also revealed a similar increase in the prevalence of HFpEF over three decades (from 41% to 56.2%). Despite the continued improvement in the survival of patients with HFrEF after the introduction of effective therapeutics, the survival of patients with HFpEF remains poor [3]. Most clinical trials of therapeutic agents, except for those of sodium-glucose cotransporter-2 inhibitors (SGLT2i), have failed to demonstrate improved survival among patients with HFpEF. This is partly because HFpEF is a heterogeneous syndrome with a variety of comorbidities [5]; thus, identifying different HFpEF phenotypes and targeting therapy based on the phenotype may be an effective strategy. Among the various subtypes of HFpEF, obesity-related HFpEF is distinct and common [6], but its diagnostic and therapeutic approaches in practice are complex. In this review, we discuss the diagnostic challenges, pathophysiology, and therapeutic options for obesity-related HFpEF using a clinical vignette.
DIAGNOSTIC CHALLENGES OF HFpEF IN OBESE INDIVIDUALS
Clinical vignette
An 80-year-old female with progressive shortness of breath during daily activities visited the outpatient clinic. She had been on antihypertensive medication for 10 years. Her body mass index (BMI) was 31.2 kg/m2 and her waist circumference was 111 cm. Computed tomography revealed prominent visceral fat (Fig. 1A). Electrocardiography revealed a normal sinus rhythm. Her initial N-terminal pro-B-type natriuretic peptide (NT-proBNP) level was 109.0 pg/mL (reference < 300 pg/mL). Coronary computed tomography angiography revealed no significant stenosis, and the calcium score was 0. Transthoracic echocardiography showed preserved left ventricular systolic function (LVEF 64%) with grade 1 diastolic dysfunction (E/A ratio 0.7, septal/lateral e’ 7.3/7.9 cm/s, average E/e’ 11.1, left atrial volume index 38.1 mL/m2, and tricuspid regurgitation [TR] Vmax 2.7 m/s) and concentric left ventricular (LV) hypertrophy (LV mass index, 106.8 g/m2; relative wall thickness, 0.48). We were unsure whether the patient fulfilled the diagnostic criteria for HFpEF and how to manage this case.
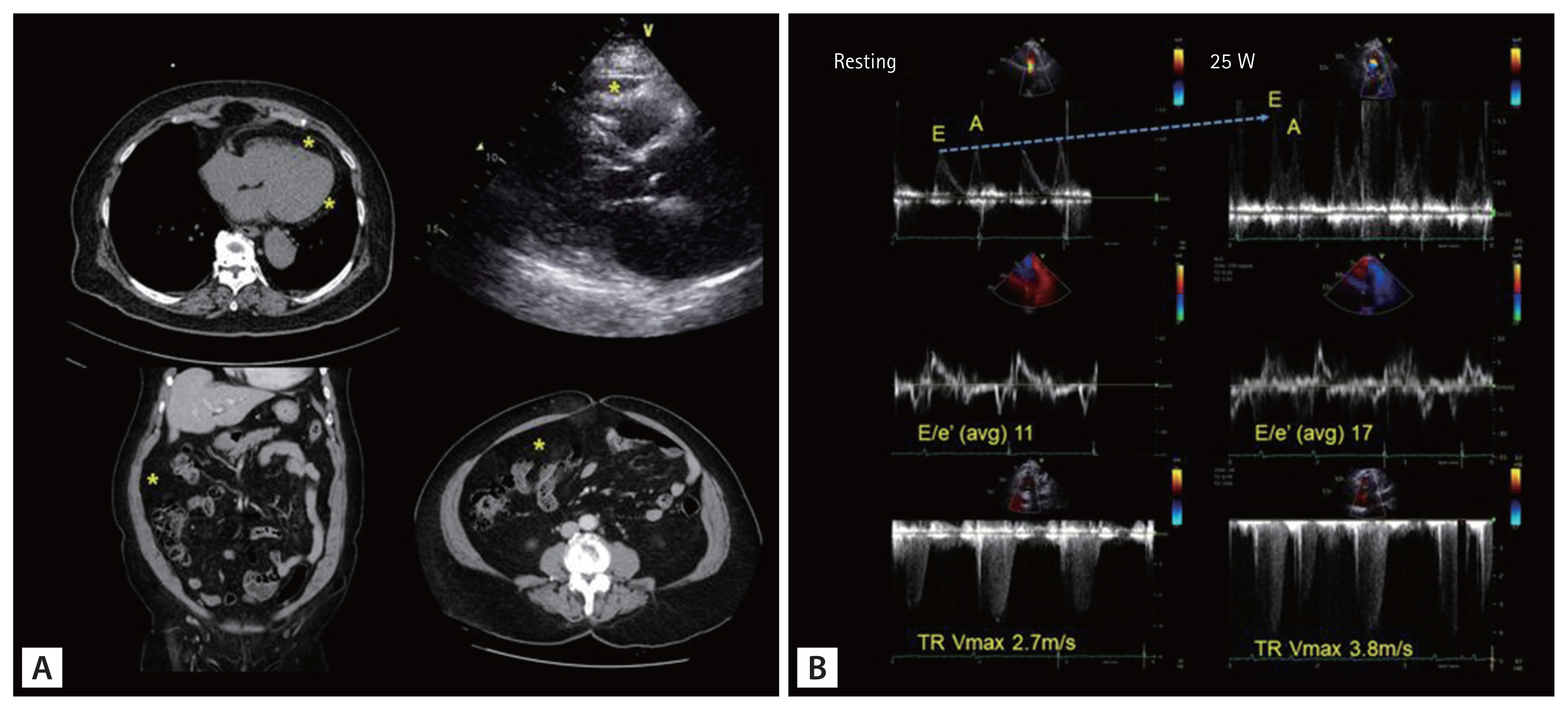
Clinical vignette of an obese woman with exertional dyspnea. (A) Note the prominent visceral fat (pericardial or mesentery fat), indicated by the yellow asterisks. (B) The usefulness of exercise echocardiography in the diagnosis of heart failure with preserved ejection fraction. The patient underwent bicycle exercise echocardiography with an initial workload of 25 W and a 25 W increase in workload every 3 minutes. She terminated exercise because of dyspnea immediately after beginning 50 W (total exercise time 3 minutes). Even under low-grade exercise (25 W), inversion of the E/A ratio and rapid elevation of the E/e’ ratio (reflecting high left ventricular filling pressure) and maximal velocity of TR were observed. TR, tricuspid regurgitation.
Diagnostic approach
Establishing an HFpEF diagnosis is challenging in patients with unexplained exertional dyspnea but no clear evidence of hypervolemia. This is particularly true in obese individuals. Although the gold standard for diagnosing HFpEF is cardiac catheterization, this method requires admission and invasive procedures, making it unsuitable for practical application in a large population. Two scoring systems (H2FPEF score and the Heart Failure Association [HFA]-PEFF score) have been suggested to facilitate HFpEF diagnosis (Fig. 2) [7,8]. Intriguingly, these two scoring systems can differentially affect the diagnosis of obesity-related HFpEF.
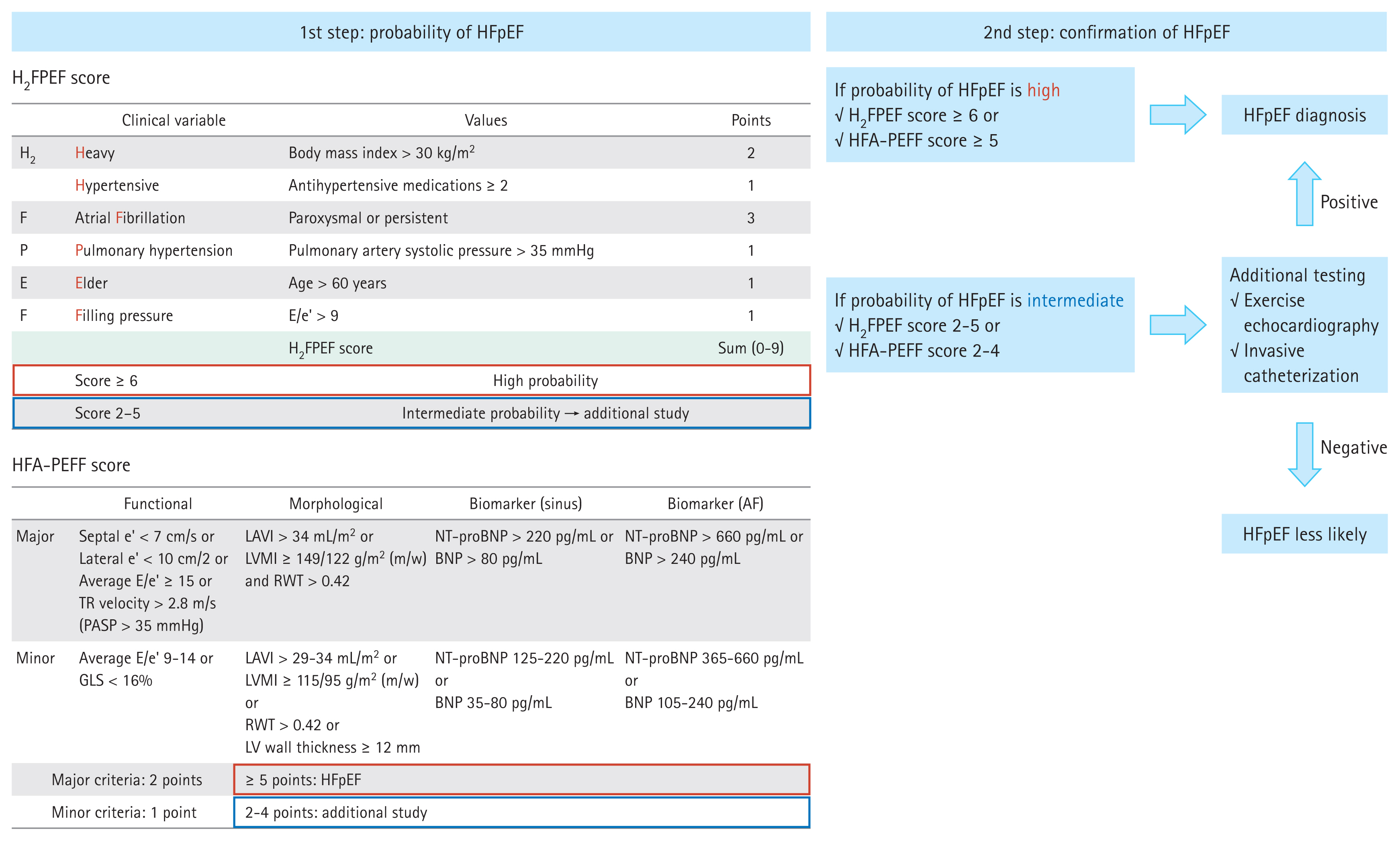
Diagnostic approach for heart failure with preserved ejection fraction. HFpEF, heart failure with preserved ejection fraction; AF, atrial fibrillation; TR, tricuspid regurgitation; PASP, pulmonary artery systolic pressure; LAVI, left atrial volume index; LVMI, left ventricular mass index; RWT, relative wall thickness; NT-proBNP, N-terminal pro-B-type natriuretic peptide; BNP, B-type natriuretic peptide; GLS, global longitudinal strain; LV, left ventricle.
First, the H2FPEF score from the Mayo Clinic is composed of four clinical parameters (obesity, hypertension, atrial fibrillation, and old age) and two echocardiographic parameters (E/e’ and pulmonary artery systolic pressure) [7]. This system does not require information about biomarkers (such as NT-proBNP). Here, obesity (defined as BMI ≥ 30 kg/m2) is allocated 2 points, increasing the possibility of HFpEF diagnosis in obese patients. Several community-based studies, including those from Asia, have demonstrated acceptable diagnostic and prognostic yields of the H2FPEF scoring system [9,10]. However, the appropriate BMI cutoff value for Asian populations, who have a lower BMI than Western populations, should be investigated separately.
Second, the HFA-PEFF score is determined based on three domains of heart function (functional, morphological, and biomarker domains) [8,11]. The HFA-PEFF score is based on nine echocardiographic parameters, which increases the complexity. The threshold NT-proBNP level is ≥ 125 pg/mL for sinus rhythm and ≥ 365 pg/mL for atrial fibrillation; however, obesity is associated with a low circulating natriuretic peptide level [6,12,13]. Thus, a caveat is needed when interpreting the HFA-PEFF score in obese individuals.
Additionally, we should consider that both scoring systems are not perfect for diagnosing HFpEF [14]. Accordingly, performing additional exercise echocardiography or invasive catheterization is mandatory in highly suspicious cases and should also be performed for obese individuals [7,8,15–17]. Many patients with HFpEF exhibit normal or marginally elevated LV filling pressure at rest (E/e’, 9–14) and only elevated LV filling pressure during exercise [15]. A recent trend in HFpEF diagnosis is to increase the sensitivity to HFpEF by lowering the LV filling pressure cutoff (resting E/e’, > 9). The latest European guidelines lowered the E/e’ cutoff from 13 to 9. Moreover, the E/e’ cutoff in the HFpEF scoring systems should be > 9 [7,8,16]. The index case patient had discordant results based on the scoring system: the H2FPEF score was 7 (confirmatory), but the HFA-PEFF score was 4 (indeterminate). Although she qualified as HFpEF based on the H2FPEF score, we planned additional confirmatory exercise echocardiography testing considering her relatively low average E/e’ and NT-proBNP levels. The E/A ratio was reversed immediately after initiating exercise (low-grade 25 W exercise), and the average E/e’ ratio increased from 11 to 17 and the pulmonary artery pressure increased from 39.2 to 67.8 mmHg (Fig. 1B). As illustrated in this case, exercise echocardiography revealed an impaired diastolic reserve during exercise, which is the primary pathophysiology of HFpEF.
A poor echocardiographic window in obese individuals is a hurdle in the diagnosis of HFpEF. Moreover, obese elderly people frequently have arthralgia when performing exercise tests, particularly a treadmill test. However, in this case, bicycle echocardiography with low-grade exercise may be a good option. Previous studies have demonstrated that rapid changes in hemodynamic parameters during the early stages of exercise (25 W, which can be performed by almost all participants) are important kinetic properties [17–19]. The recommended exercise echocardiographic evidence for elevated LV filling pressure is peak E/e’ ≥ 15 and/or peak TR velocity > 3.4 m/s according to the 2021 European Society of Cardiology heart failure guidelines [16]. However, various exercise echocardiographic parameters with different cut-offs (septal E/e’ > 15 or average E/e’ > 14 and peak TR velocity > 2.8 m/s) have been suggested by other guidelines and studies [16,17,20]. Therefore, further studies are needed to verify the appropriate diagnostic criteria for HFpEF based on obesity and ethnicity.
PATHOPHYSIOLOGY OF OBESITY-RELATED HFpEF
Obesity contributes to the occurrence of HFpEF via multiple pathways (Fig. 3). Among them, sodium retention and systemic inflammation are the main drivers of HFpEF in individuals with obesity. Renal tubular sodium retention and plasma volume expansion occur in obese people due to the overproduction of aldosterone and enhanced degradation of natriuretic peptides [6,21–26]. Adipocytes directly synthesize aldosterone and indirectly enhance aldosterone secretion from the adrenal gland by releasing leptin and catecholamines [25,26]. Adipocytes are also associated with increased expression of neprilysin, which enhances the degradation of natriuretic peptides, which in turn increases the lelvel of aldosterone. Adipose tissue in obese individuals undergoes a shift to a pro-inflammatory state, which contributes to the stiffening of the heart and vessels via extracellular matrix remodeling, thrombosis, and inflammation. Unlike subcutaneous fat (which is often regarded as quiescent fat), visceral fat, particularly that surrounding the heart and vessels, exerts deleterious effects by secreting pro-inflammatory adipokines. Among the different visceral fat types, epicardial adipose tissue is currently considered an important target in HFpEF [27–29]. Beyond its inflammatory action, epicardial and pericardial fat inhibits cardiac distensibility by acting as a mechanical restraint [6]. Obesity is commonly associated with comorbidities, such as hypertension, diabetes, and obstructive sleep apnea, all of which can lead to HFpEF.
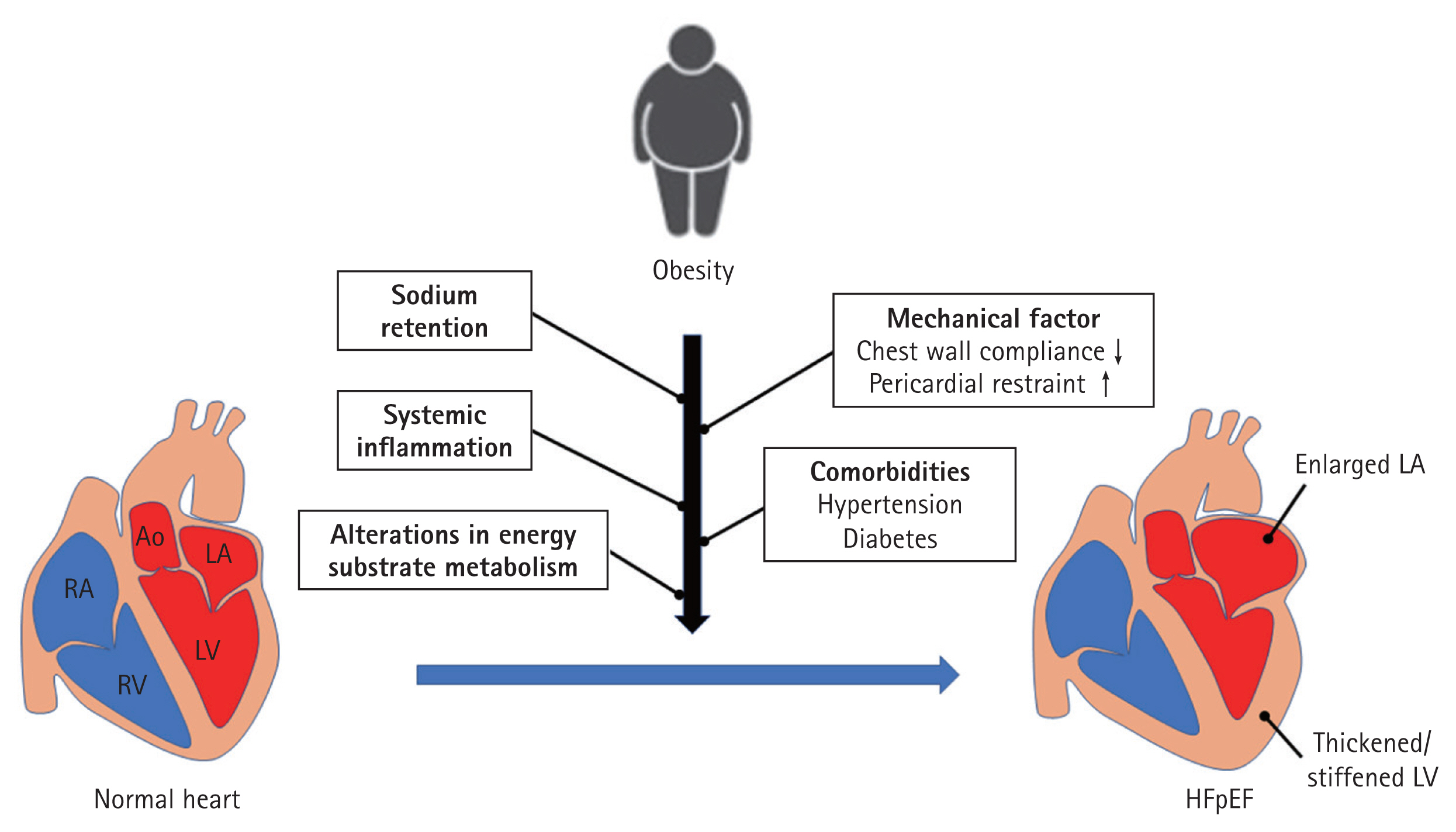
Pathophysiology of obesity-related heart failure with preserved ejection fraction. RA, right atrium; RV, right ventricle; Ao, aorta; LA, left atrium; LV, left ventricle; HFpEF, heart failure with preserved ejection fraction.
The heart can accommodate the expanded volume by dilating the LV under normal conditions. However, a stiffened heart due to inflammation in the case of obese HFpEF cannot adequately accommodate the expanded volume due to the obesity, ultimately resulting in elevated LV filling pressure, even at low levels of physical activity [24]. In particular, obesity is closely related to increased LV filling pressure in women [28,30–32]. The exact mechanisms behind the sex-specific association remain unclear. However, fat-pre-dominant body composition (higher fat at a given BMI) and higher LV filling pressure, even with the same amount of fat, in females may be causes. Further studies of female pre-dominance in obese patients with HFpEF are needed.
THERAPEUTIC OPTIONS
Lifestyle modifications: caloric restriction and exercise
Several studies have reported the benefits of caloric restriction and exercise on LV diastolic function and/or exercise capacity [33–35]. In a small, well-designed randomized clinical trial, Kitzman et al. [34] demonstrated that caloric restriction or aerobic exercise increases exercise capacity (expressed as peak VO2), and the effect of caloric restriction and aerobic exercise may be synergistic in older obese patients with stable HFpEF. However, the effect of weight loss may be transient, and weight gain after intentional weight loss (the so-called yo-yo effect) is frequent in the real world. Therefore, a more predictable and sustainable approach to weight control (either medical or surgical) is required.
Bariatric surgery
Most obese Asian patients with HFpEF (corresponding BMI 25–35 kg/m2), whom we frequently encounter in practice, may not be candidates for bariatric surgery. However, HFpEF with severe obesity (BMI ≥ 40 kg/m2) is frequently observed in Western countries [36–38]. In one study, bariatric surgery alleviated exertional dyspnea and leg edema in heart failure patients with severe obesity (median BMI, 55 kg/m2) [36]. One study included only obese women with HFpEF (mean BMI, 43.9 kg/m2) and found that bariatric surgery was associated with weight loss, improved symptoms, reverse heart remodeling, and improved cardiac relaxation [38].
Medications that ameliorate the adverse effects of obesity on the heart
Although the diagnosis of HFpEF can be obtained via complex process and test, the available treatment options are limited. However, according to the results of The Empagliflozin Outcome Trial in Patients with Chronic Heart Failure with Preserved Ejection Fraction (EMPEROR-Preserved) [39] and Dapagliflozin Evaluation to Improve the LIVEs of Patients With PReserved Ejection Fraction Heart Failure (DELIVER) [40] trials, SGLT2i should be considered in individuals with HFpEF. The salutary effects of SGLT2i appear to be similarly applicable to those of obese and non-obese HFpEF patients [39,40]. Despite the distinct benefit to heart failure, the mechanism behind how SGLT2i lead to cardiovascular (CV) benefits is not fully understood. Considering the early CV benefit that occurs within 1 month, pleiotropic effects, beyond the glucose-lowering effect, are gaining more attention [41–43]. SGLT2i modulate two main pathophysiologies of obesity-related HFpEF (sodium retention and systemic inflammation) via hemodynamic and metabolic pathways (Fig. 4). The natriuresis-driven effect is the mainstay from a hemodynamic perspective and is thought to also play an important role in obese HFpEF by addressing obesity-related sodium retention. Decreased sodium reabsorption by SGLT2i leads to an increased sodium concentration in the macular densa, which activates tubuloglomerular feedback and constricts the afferent glomerular arterioles. Consequently, there is a decrease in intraglomerular pressure and renal protection. Additionally, natriuresis is associated with decreased plasma volume (approximately 7% reduction) and blood pressure (4/2 mmHg reduction in systolic/diastolic blood pressure), which can be translated into decreased preload and afterload of the heart [42–46]. SGLT2i-induced glucosuria is another important axis in resolving HFpEF pathophysiology. SGLT2i are associated with a negative caloric balance via the excretion of 200–250 kcal/day in the urine, which reduces body weight (approximately 2–3 kg) [44,46]. Weight loss with SGLT2i use appears to be accompanied by a reduction in body fat and extracellular fluid but does not affect lean mass [47]. Furthermore, a lower glucose level decreases insulin resistance and enhances the secretion of glucagon, leading to lipolysis in adipose tissue. The increased free fatty acids are taken up by the liver, where they undergo oxidation and are converted to acetyl-CoA and ketone bodies. The high ketone body production and metabolism favorably contribute to CV and renal outcomes by acting as an efficient energy substrate and improving mitochondrial respiration (adenosine triphosphate hydrolysis), although this hypothesis warrants further studies [48–54]. Moreover, these metabolic changes help alleviating obesity-related inflammation and fibrosis [50–53]. Taken together, these findings beneficially affect the kidney and heart individually and via cardio-renal interaction.
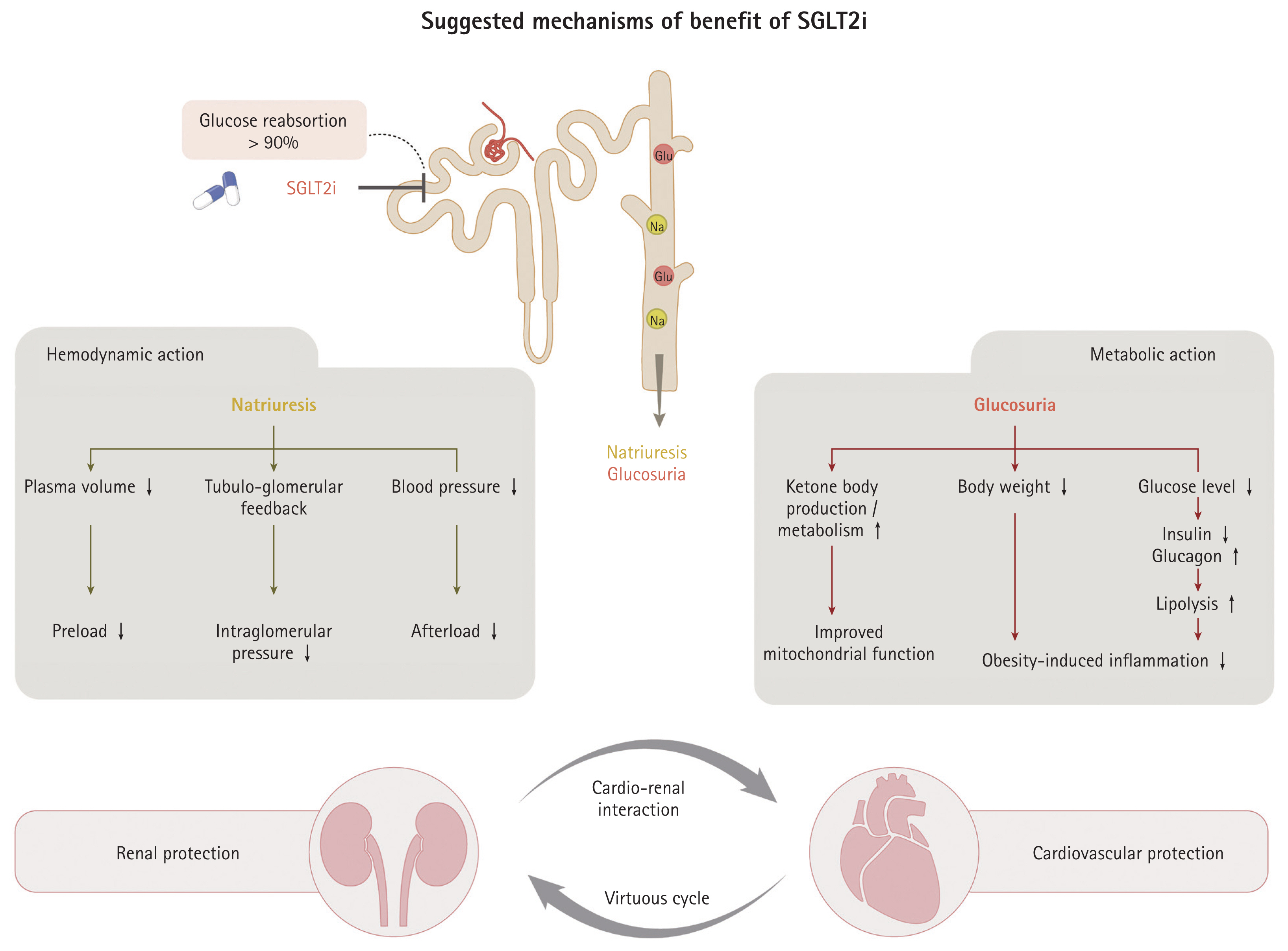
The postulated mechanism of the benefit of sodium-glucose cotransporter 2 inhibitors (SGLT2i) for obesity-related heart failure with preserved ejection fraction. The effects of SGLT2i according to their hemodynamic and metabolic actions are summarized. Collectively, these mechanisms synergistically and positively affect kidney and heart outcomes. Furthermore, cardio-renal interactions that lead to a virtuous cycle are linked to a favorable outcome. The overall effect of SGLT2i appears to be similar between obese and non-obese individuals with heart failure with preserved ejection fraction.
Considering that sodium retention and high aldosterone levels are frequently found in obesity-related HFpEF, mineralocorticoid receptor antagonists (MRAs) may be a good therapeutic option. Among the diverse HFpEF phenotypes, MRAs are associated with reduced risks of CV death, hospitalization for heart failure, and aborted cardiac arrest in patients with obesity-related HFpEF [55]. In the TOPCAT trial (Aldosterone Antagonist Therapy for Adults with Heart Failure and Preserved Systolic Function), subgroup analyses according to BMI category revealed a significant risk reduction in the primary endpoints (CV death and hospitalization for heart failure) in the obese groups (hazard ratio [HR], 0.80; 95% confidence interval [CI], 0.66–0.98) [56]. Moreover, the effect of obesity on the response to spironolactone in patients with HFpEF was explicitly explored in post hoc analyses of the TOPCAT American cohort [57]. In that study, obesity was defined by either BMI or waist circumference, and spironolactone was associated with a significant risk reduction in CV death or hospitalization for heart failure in obese patients but not in non-obese subjects (HR, 0.618; 95% CI, 0.460–0.831 in those with BMI ≥ 30 kg/m2 compared with their counterparts; HR, 0.740; 95% CI, 0.559–0.980 in those with a large waist circumference compared with their counterparts). However, further randomized trials evaluating obese HFpEF are required to clarify the effect of MRAs on clinical outcomes.
Neprilysin inhibitors are expected to have beneficial effects, given the deficiency of natriuretic peptides in patients with obesity-related HFpEF. However, the PARAGON-HF trial reported no significant CV benefit of sacubitril-valsartan over valsartan in HFpEF subjects [58,59]. The treatment interaction based on sex and LVEF is controversial. Furthermore, that study was designed to compare the effect of sacubitril-valsartan against an active comparator (valsartan), which may have affected the results. A putative placebo analysis showed the potential benefit of sacubitril–valsartan compared with a putative placebo (HR, 0.71; 95% CI, 0.54–0.93) [60]. Individuals with severe obesity (BMI > 40 kg/m2) were excluded at enrollment, which limited the conclusions that could be drawn regarding the drug effect in obese patients with HFpEF.
A summary of recent clinical trials of these three medications in obesity-related HFpEF is presented in Table 1. It is important to remember when interpreting the results of recent HFpEF clinical trials that the inclusion criteria for HFpEF differed somewhat from the definition of HFpEF stated in guidelines and/or the characteristics of real-world HFpEF patients. Indeed, a substantial number of patients with a LVEF of 40–49% (which falls into the heart failure with mildly reduced EF category) were enrolled in the study. Furthermore, more advanced-stage HFpEF (history of hospitalization) and less obese patients may have been preferentially included in the study to meet the requirement of an elevated NT-proBNP. Subsequent subgroup-specific randomized clinical and prospective cohort studies are required to clarify the effects of these drugs.
CONCLUSIONS
Obesity-related HFpEF is a distinct entity of HFpEF with a unique pathophysiology. However, decreased natriuretic peptide levels and a poor echocardiographic window in obese patients often hamper the diagnosis. Exercise stress echocardiography may be helpful when the HFpEF diagnosis is uncertain. There are promising new drugs for HFpEF, although additional clinical trials are needed to identify the best combination of medications and determine how these drugs can modulate the pathophysiology of obesity-related HFpEF.
Notes
No potential conflict of interest relevant to this article was reported.