Urgent need for novel antibiotics in Republic of Korea to combat multidrug-resistant bacteria
Article information
Abstract
Multidrug resistance in bacteria is an important issue and is increasing in frequency worldwide because of the limitations of therapeutic agents. From 2010 to 2019, 14 new systemic antibiotics received regulatory approval in the United States. However, few new antibiotics have been introduced in Republic of Korea to combat multidrug-resistant pathogens. Here, we introduce six novel antibiotics for Gram-positive bacteria and five for Gram-negative bacteria approved by the United States Food and Drug Administration and the European Medicines Agency from 2009 to October 2021, and recommend that they be approved for use in Republic of Korea at the earliest possible date.
INTRODUCTION
Multidrug-resistant (MDR) bacteria is a critical problem that is on the increase worldwide because of limitations in available therapeutic agents [1–3]. Consequently, the development of new antibiotics is a matter of urgency. From 2010 to 2019, 14 new systemic antibiotics received regulatory approval in the United States [4,5]. In Republic of Korea, MDR bacteria are prevalent not only in nosocomial infections but also in community-acquired infections [6–11]. The Korea Disease Control and Prevention Agency designated vancomycin-resistant Staphylococcus aureus (VRSA) and carbapenem-resistant Enterobacterales (CRE) as group 2 nationally notifiable infectious diseases, and methicillin-resistant S. aureus (MRSA), vancomycin-resistant Enterococcus (VRE), multidrug-resistant Pseudomonas aeruginosa (MRPA), and multidrug-resistant Acinetobacter baumannii (MRAB) as group 4 nationally notifiable infectious diseases [12]. According to the Korean global antimicrobial resistance surveillance system, 53.2% of S. aureus blood strains in Republic of Korea were MRSA and 34% of Enterococcus faecium strains were VRE in 2017 [13]. In 2015, 85% of A. baumannii and 35% of P. aeruginosa were resistant to imipenem [12]. The prevalence of CRE infections has rapidly increased [14]. In Republic of Korea, the challenges associated with obtaining approval for new drugs complicates treatment of infections caused by MDR bacteria. These challenges include the high cost of new antibiotics, making them unaffordable for the domestic health insurance system. Antibiotics introduced in Republic of Korea over the past decade include tigecycline (2007), doripenem (2010), zabofloxacin (2015), and daptomycin (2021), which have been approved of the Ministry of Food and Drug Safety (MFDS). Tedizolid (2015) was approved but not sold in Republic of Korea, and ceftolozane/tazobactam (2017) was approved by the MFDS as non-reimbursable [15]. Here we introduce novel antibiotics for MDR bacteria that have received regulatory approval by both the U.S. Food and Drug Administration (FDA) and the European Medicines Agency (EMA) from 2009 to October 2021. We recommend that these antibiotics be adopted in Republic of Korea. The scope of the review did not encompass new drugs targeting tuberculosis, Clostridioides difficile, fungi, or viruses.
AGENTS TARGETING GRAM-POSITIVE BACTERIA
Table 1 lists novel antibiotics active against Gram-positive bacteria that were approved by the FDA and EMA from 2009 to October 2021.
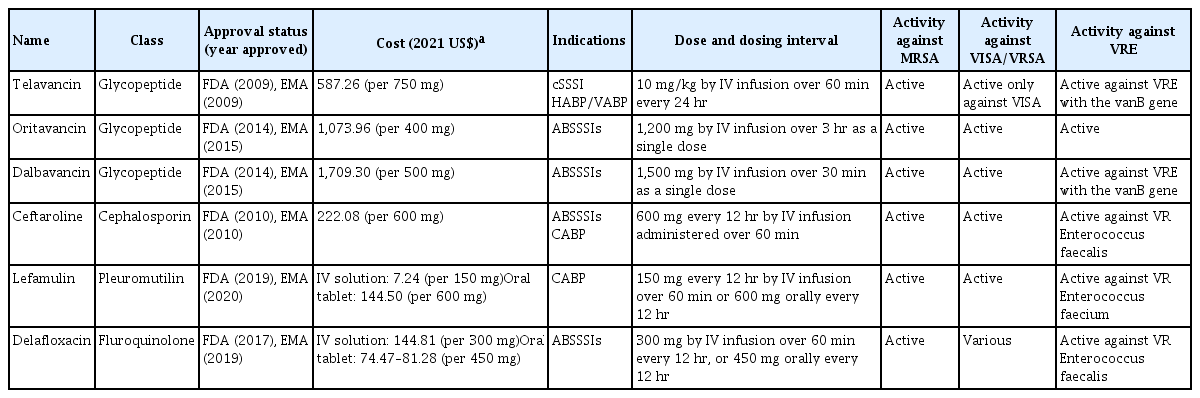
Characteristics of new Gram-positive antibacterial drugs that received regulatory approval by the FDA and EMA from 2009 to October 2021
Telavancin
Telavancin, oritavancin, and dalbavancin are next-generation lipoglycopeptide antibiotics. They are vancomycin derivatives that contain both lipophilic and hydrophobic side chains. This structural modification enhances their antibacterial activity compared to vancomycin [16].
Telavancin is effective for the treatment of Gram-positive bacterial infections, especially MRSA [17]. Although vancomycin and telavancin have similar activities, telavancin has a longer half-life and need be administered only once a day, and it has better tissue permeability than vancomycin [18]. In a clinical study of complicated skin and skin structure infections, telavancin was comparably effective to standard of care, such as vancomycin [19,20]. The rate of microbiological eradication in patients with MRSA infections was 84% in the telavancin group and 74% in the standard of care group. Telavancin exhibited noninferiority to vancomycin in two phase 3 clinical trials involving patients with nosocomial pneumonia caused by Gram-positive bacteria [21]. However, when telavancin was used in patients with impaired renal function (creatinine clearance rate ≤ 50 mL/min), the mortality rate was higher than that of vancomycin. Therefore, clinicians should consider using telavancin instead of vancomycin for the treatment of MRSA in cases with a high minimum inhibitory concentration of vancomycin. The risks and benefits to patients should be weighed when making treatment decisions.
Enterococci harboring the vanA gene can be resistant to telavancin; however, enterococci with the vanB gene are susceptible. Therefore, telavancin can be used to treat infections caused by VRE strains that have the vanB gene [17].
Oritavancin
Oritavancin is a novel lipoglycopeptide antibiotic active against MRSA, VRSA, and VRE [16]. Oritavancin has good antimicrobial activity against VRE. The minimum inhibitory concentration of oritavancin for 90% of strains was 0.12 μg/mL for VRE that harbor vanA, and ≤ 0.008 μg/mL for VRE that harbor the vanB gene [16].
Oritavancin was approved by the FDA for the treatment of acute bacterial skin and skin structure infections (ABSSSIs). Oritavancin has a long half-life (393 hours), and a single dose of 1,200 mg is recommended for ABSSSIs. A randomized trial of oritavancin revealed its noninferiority to vancomycin for the treatment of ABSSSIs [22]. The investigator-assessed clinical cure rate was 79.6% in the oritavancin group and 80.0% in the vancomycin group.
Dalbavancin
Dalbavancin was developed as an alternative to vancomycin; its higher antimicrobial activity and prolonged half-life compared with vancomycin enable administration of dalbavancin as a single dose treatment regimen for ABSSSIs [23]. A randomized clinical trial demonstrated its noninferiority to vancomycin followed by oral linezolid for ABSSSIs (response rate, 79.7% vs. 79.8%; 95% confidence interval [CI] of the weighted difference, −4.5 to 4.2) [24]. Additionally, dalbavancin was approved by the FDA for the treatment for ABSSSIs in pediatric patients from birth [25]. Dalbavancin reportedly exhibits satisfactory activity against MRSA and even biofilms of S. aureus. It also has activity against VRE with the vanB gene but not strains with the vanA gene [26].
Ceftaroline
As for other β-lactams, ceftaroline binds to penicillin-binding proteins to inhibit cell-wall synthesis. Ceftaroline exhibits a high affinity for penicillin-binding protein 2a, which is related to methicillin resistance [27]. Ceftaroline can be used for the treatment of infections caused by MRSA, vancomycin intermediate-resistant S. aureus (VISA), and VRSA. It has in vitro activity against vancomycin-resistant Enterococcus faecalis but not against E. faecium. In addition, ceftaroline has activity against clinically relevant Gram-negative bacteria, including Haemophilus influenzae, Moraxella catarrhalis, non-extended-spectrum β-lactamase (ESBL)-producing Escherichia coli, and Klebsiella pneumoniae but not against P. aeruginosa or Acinetobacter species or β-lactamase-producing, AmpC-derepressed Enterobacterales [27,28].
In phase 3 studies, the clinical cure rate of ceftaroline was noninferior to that of vancomycin plus aztreonam (91.6%–92.2% vs. 92.1%–92.7%) for ABSSSIs [29–32]. Phase 3 trials involving patients with community-acquired bacterial pneumonia (CABP) indicated that ceftaroline was noninferior to ceftriaxone [33]. The clinical cure rate for ceftaroline and ceftriaxone was 84.3% and 77.7%, respectively.
Lefamulin
Lefamulin was the first systemic pleuromutilin antibiotic agent approved by the FDA and EMA, and is available as oral and intravenous (IV) formulations [34]. It has broad-spectrum activity against Gram-positive, Gram-negative, and anaerobic pathogens, as well as atypical bacteria [35]. Lefamulin has bactericidal activity against MRSA and vancomycin-resistant E. faecium but not E. faecalis [35,36]. H. influenzae and M. catarrhalis are reportedly susceptible, and lefamulin has limited activity against P. aeruginosa, A. baumannii, and Enterobacterales [34,35].
For the treatment of CABP, lefamulin (oral and IV formulations) was noninferior to moxifloxacin and well tolerated [37,38]. Additionally, a phase 2 trial demonstrated the efficacy of lefamulin against ABSSSIs [39].
Delafloxacin
Delafloxacin is a fluoroquinolone with increased activity in acidic environments, such as those encountered with ABSSSIs. It is active against most Gram-positive pathogens, including MRSA and E. faecalis [40]. When used to treat MRSA biofilm-mediated infections, delafloxacin was more potent than daptomycin [41]. However, delafloxacin was active against only 4.6% of E. faecium strains [42]. In addition, 40% of VISA isolates were susceptible to delafloxacin; however, only 7% of VRSA isolates were susceptible [43]. Regarding Gram-negative bacteria, most fluoroquinolone-resistant Enterobacterales in one study had lower susceptibility to delafloxacin than susceptible Enterobacterales [44]. Only 54% of P. aeruginosa isolates were susceptible to delafloxacin [42].
Delafloxacin is reportedly noninferior to vancomycin plus aztreonam for the treatment of ABSSSIs [45,46], and is safer and more efficacious than tigecycline [47]. Compared with moxifloxacin, delafloxacin was effective against CABP [48]. In seven hospitals in New York City, 22% of MRSA isolates were resistant to delafloxacin [49]. Therefore, it is important to determine the delafloxacin susceptibility of MRSA strains isolated in Republic of Korea.
AGENTS TARGETING GRAM-NEGATIVE BACTERIA
Table 2 lists the novel antibiotics active against MDR Gram-negative bacteria approved by the FDA and EMA from 2009 to October 2021. Their activities against MDR Gram-negative bacteria are described in Table 3.
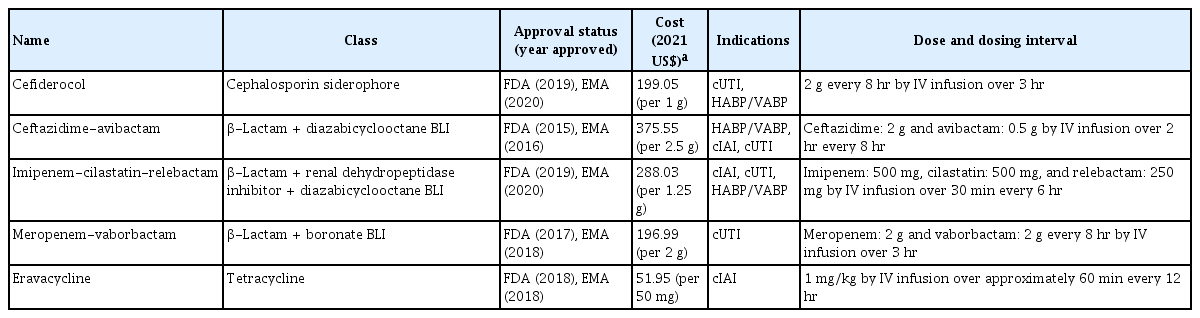
Characteristics of new Gram-negative antibacterial drugs that received regulatory approval by the FDA and EMA from 2009 to October 2021
Cefiderocol
Cefiderocol was the first novel siderophore cephalosporin approved for MDR Gram-negative pathogens. It has a unique iron transporter-based mechanism that uses the siderophore–iron complex pathway of Gram-negative bacteria to enable penetration of the bacterial outer membrane [50]. Cefiderocol is active against all Ambler classes of β-lactamases (e.g., K. pneumoniae carbapenemase [KPC], Verona integron-encoded metallo-β-lactamase, oxacillinase [OXA]-48-like carbapenemases), and New Delhi metallo-β-lactamase (NDM) [51,52]. It also has considerable activity against MRAB, MRPA, and Stenotrophomonas maltophilia [52–56].
In a phase 2 study, cefiderocol was noninferior to imipenem-cilastatin for patients with complicated urinary tract infections (cUTIs) caused by Gram-negative bacteria [57]. In a phase 3 study of treatments for nosocomial pneumonia caused by Gram-negative pathogens, cefiderocol was noninferior to high-dose, extended-infusion meropenem [58]. In another phase 3 study, its efficacy and safety for the treatment for serious infections caused by carbapenem-resistant Gram-negative bacteria were confirmed in comparison to the best-available therapy. However, there were more deaths in the cefiderocol group than in the best available therapy group (34% vs. 18%) by the end of the study period, particularly in patients with Acinetobacter spp. infections [59]. Resistance to cefiderocol has been reported [60,61].
Ceftazidime-avibactam
Ceftazidime-avibactam is a combination of a third-generation cephalosporin and a newly developed non-β-lactam β-lactamase inhibitor, prepared at a fixed ceftazidime: avibactam ratio of 4:1 [62]. It has high activity against ESBL-, AmpC-, KPC-, and OXA-48-producing Enterobacterales. Moreover, MRPA is susceptible to ceftazidime-avibactam [52]. However, the drug is not active against most Acinetobacter species or metallo-β-lactamase-producing isolates [63].
Ceftazidime-avibactam plus metronidazole exhibited noninferiority to meropenem in a phase 3 study of patients with complicated intraabdominal infections [64]. Ceftazidime-avibactam was noninferior to doripenem for the treatment of cUTIs [65]. The microbiological eradication rate was 77.4% in the ceftazidime-avibactam group and 71.0% in the doripenem group (95% CI for the difference, 0.33% to 12.36%). Ceftazidime-avibactam exhibited high activity against KPC-producing CRE compared to colistin in a prospective observational study [66]. It is also reportedly noninferior to meropenem for the treatment of nosocomial pneumonia and ventilator-associated pneumonia [67].
Because of the limited availability of therapeutic agents, ceftazidime-avibactam has emerged as an alternative to colistin for the treatment of infections caused by CRE [68,69]. Ceftazidime-avibactam resistance has been reported in carbapenem-resistant K. pneumoniae isolates [70–72]. Therefore, the application of and rationale for the use of ceftazidime-avibactam require careful consideration [69,73].
Imipenem-cilastatin-relebactam
Imipenem-cilastatin-relebactam is a combination of a pre-existing carbapenem, imipenem-cilastatin, and a new β-lactamase inhibitor, relebactam. It has activity against KPC-producing, but not NDM-producing, Enterobacterales. It is also active against MRPA [52]. However, imipenem-cilastatin-relebactam has limited activity against A. baumannii isolates that harbor class D β-lactamases [74].
Imipenem-cilastatin-relebactam reportedly exhibits noninferiority to piperacillin/tazobactam in patients with nosocomial pneumonia and ventilator-associated pneumonia, including those at high risk [75,76]. In a phase 2 study involving patients with cUTIs, imipenem-cilastatin-relebactam (125 mg relebactam) was noninferior to imipenem-cilastatin (clinical response rate 97.1% vs. 98.8%) [77]. Imipenem-cilastatin-relebactam also yields favorable clinical responses in patients with imipenem-non-susceptible bacterial infections and has a lower all-cause mortality rate than colistin plus imipenem-cilastatin [78].
Meropenem-vaborbactam
Meropenem-vaborbactam is the first approved combination of a carbapenem and a β-lactamase inhibitor. Meropenem-vaborbactam is effective against numerous Gram-negative bacteria, including KPC-producing CRE [52]. However, vaborbactam has no activity against NDM and OXA-48-like producers and does not improve the activity of meropenem against non-fermenting Gram-negative bacteria, such as carbapenem-resistant P. aeruginosa or Acinetobacter spp. [79]. In patients with cUTIs, meropenem-vaborbactam showed an overall treatment success rate of 98.4% in a randomized clinical trial and was noninferior to piperacillin-tazobactam (overall success rate, 94.0%; 95% CI for the difference, 0.7% to 9.1%) [80]. In a study of CRE infections, meropenem-vaborbactam had a lower mortality rate, a higher clinical cure rate, and less nephrotoxicity than the best available therapy [81].
Eravacycline
Eravacycline is a novel fluorocycline antibiotic with a similar structure to tigecycline; however, eravacycline is more potent against Gram-positive, Gran-negative, and anaerobic pathogens than tigecycline. Specifically, it is active against MRSA, VRE, ESBL-producing Enterobacterales, CRE, and MRAB. However, as for tigecycline, eravacycline has no activity against P. aeruginosa, Morganella spp., Proteus spp., or Providencia spp. [52,82].
In a phase 3 study of patients with complicated intraabdominal infections, eravacycline exhibited noninferiority to meropenem (clinical cure rate, 90.8% vs. 91.2%; 95% CI, −6.3% to 5.3%) [83]. A randomized clinical trial compared eravacycline and levofloxacin for the treatment of cUTIs; however, to our knowledge, the results have not been published [84]. Based on available data, eravacycline is a potential alternative to conventional antibiotics for the treatment of infections caused by MDR bacteria [69]. However, it should be used with caution to avoid the emergence of resistance [85].
CONCLUSIONS
Infections with MDR bacteria have high mortality rates [86,87]. Currently, few antibiotics are available in Republic of Korea. In addition to those covered in this review, other antibiotics—such as iclaprim, ceftobiprole medocaril, aztreonam-avibactam, and darobactin—await regulatory approval by the FDA and EMA. To prevent the emergence of antibiotic resistance, new antibiotics should not be used empirically; they should be based on the susceptibility of and resistance genes harbored by the causative bacteria. Rapid identification of pathogens and resistance genes is essential for effective antibiotic treatment [88]. In addition, antimicrobial stewardship programs should be implemented in hospitals [89,90]. The Republic of Korean national action plan on antimicrobial resistance was established in 2016. Collaborative efforts by the government, academia, and pharmaceutical companies would expedite the regulatory approval of novel antibiotics in Republic of Korea, enhancing patient care and treatment.
Notes
No potential conflict of interest relevant to this article was reported.