Anti-fibrotic effects of branched-chain amino acids on hepatic stellate cells
Article information
Abstract
Background/Aims
Patients with liver cirrhosis (LC) have low levels of branched-chain amino acids (BCAAs). There is accumulating evidence that BCAAs have anti-fibrotic effects in cirrhosis. This study is aimed to evaluate the effect of BCAAs on the function and phenotype of activated hepatic stellate cells (HSCs).
Methods
LX-2, an immortalized human stellate cell line, was used in in vitro experiments. LX-2 cells were exposed to transforming growth factor β1 (TGF-β1) and BCAAs or to valine, leucine, and isoleucine, which are components of BCAAs. Activation of the TGF-β signaling pathway in LX-2 cells was observed using real-time quantitative polymerase chain reaction and Western blotting.
Results
The increased expression of snail family transcriptional repressor 1 (SNAI1) was observed in LX-2 cells activated by TGF-β1. After BCAA treatment, its expression was significantly decreased at the mRNA level. The increased expression of Col1α1 and TIMP2 at the mRNA level and alpha smooth muscle actin at the protein level in activated LX-2 cells decreased after BCAA treatment. Among the BCAA components, leucine and valine significantly abrogated TGF-β-induced activation of LX-2 cells. BCAA treatment led to the decreased phosphorylation of Smad2 and p38 proteins, which are markers for Smad and Smad-independent p38 mitogen-activated protein kinase signaling pathways, respectively.
Conclusions
BCAA treatment can improve hepatic fibrosis by directly affecting the activated state of hepatic stellate cells through inhibition of the TGF-β signaling pathway. Among BCAA components, leucine and valine mainly abrogated TGF-β-induced activation of HSCs. Our results suggest that BCAA may be used to attenuate the progression of liver fibrosis.
INTRODUCTION
Liver cirrhosis (LC) is a disease of global concern, responsible for more than one million worldwide deaths in 2010 [1]. Although LC was previously considered to be irreversible, there is overwhelming evidence that regression of fibrosis is possible with therapy that targets specific causes of cirrhosis, such as potent anti-viral therapies for viral hepatitis and abstinence of alcohol. In addition, many researchers have focused on the process of fibrogenesis to develop anti-fibrotic therapies.
Hepatic fibrosis means the accumulation process of extracellular matrix (ECM) proteins, and hepatic stellate cells (HSCs), which reside in the space of Disse, are the main producers of ECM proteins. Following liver injury, HSCs undergo transdifferentiation from quiescent cells into fibrogenic, proliferative, and contractile myofibroblast-like cells, characterized by expression of alpha smooth muscle actin (α-SMA) [2]. Transforming growth factor-β (TGF-β), the most predominant fibrogenic cytokine, is secreted from several cells including HSCs in the liver during disease conditions and activates HSCs to produce ECM proteins including type I and III collagen and fibronectin, leading to hepatic fibrosis [3]. Thus, controlling HSC activation is considered a promising target of anti-fibrotic therapies.
Branched-chain amino acids (BCAAs) are composed of valine, leucine, and isoleucine and are essential for synthesis of body proteins while also exerting inhibitory effects on protein degradation [4]. In LC patients, the serum ratio of BCAAs to aromatic amino acids (AAAs) is characteristically low under several conditions, such as detoxification of ammonia in skeletal muscle or insufficient nutritional intake. Therefore, previous researchers tried to identify whether BCAA supplementation could improve the prognosis of LC patients. Supplementation with BCAAs had beneficial effects on hepatic encephalopathy without adverse events, mainly by enhancing the ratio of BCAAs to AAAs. Thus, recent guidelines recommend oral administration of BCAAs for such patients.
In addition, it has been suggested that BCAA supplementation had beneficial effects including improvement of insulin sensitivity, regression of fibrosis, and reduced incidence of hepatocellular carcinoma (HCC) in LC patients [5,6]. In a previous study, we showed that BCAA treatment produced anti-cancer and anti-fibrotic effects in a rat model with LC and HCC induced by diethylnitrosamine. The BCAA-treated group showed down-regulation of fibrosis markers, such as TGF-β1 and collagen, at both mRNA and protein levels. Expression of angiogenesis and apoptosis inhibitor markers also decreased with BCAA treatment, which could lead to suppression of HCC development [7].
However, most of the studies that demonstrated a protective effect of BCAAs against hepatic fibrosis were population-based cohorts or in vivo experiments. Thus, it is unclear as to the detailed mechanism of BCAA treatment in the process of fibrogenesis. As HSCs are central to the process of hepatic fibrosis, we reasoned that BCAAs would have a role in controlling activation of HSCs. To address this, we used LX-2 cells, a human HSC line, and evaluated the direct role of BCAAs in LX-2 cells. We also analyzed the individual effect of each component of BCAAs in LX-2 cells to determine which had the strongest effect on fibrosis regression.
METHODS
Chemicals and reagents
Recombinant human TGF-β1 (240-B) was purchased from R&D Systems (Minneapolis, MN, USA). The BCAA components (valine, leucine, and isoleucine) and amino acid-free medium (ZERO medium) were obtained from Ajinomoto Pharmaceuticals Co. (Tokyo, Japan) (Table 1). BCAA mixture (leucine/isoleucine/valine = 2:1:1.2) was freshly dissolved in nuclease-free water (Ambion, AM9930) at 100 mmol/L.
Cell culture and treatment
Human HSCs (LX-2, MilliporeSigma, Burlington, MA, USA) were maintained in Dulbecco’s modified Eagle’s medium (DMEM) supplemented with 10% fetal bovine serum and 1% antibiotic-antimycotic. Low-amino acid medium (× 1/2 DMEM) was prepared by diluting DMEM with ZERO medium. We evaluated the effects of BCAAs on LX-2 cells cultured in low-amino acid medium to create an environment for LX-2 cells similar to that of HSCs in a cirrhotic liver. After incubation for 24 hours, LX-2 cells were transferred to low-amino acid medium and starved for 24 hours. Then, the cells were treated with different concentration (5, 10, and 20 mM) of BCAAs or each component of BCAAs, leucine, isoleucine and valine for 1 hour before the addition of TGF-β1 (2.5 ng/mL). After incubation for 48 hours, the cells were harvested for analysis.
RNA extraction, real-time quantitative polymerase chain reaction, and semi-quantitative reverse transcription polymerase chain reaction
Total RNA was extracted using Trizol reagent (Thermo Fisher Scientific, Basingstoke, UK), and 1 μg of total RNA was reverse transcribed using the QuantiTect Reverse Transcription Kit (Qiagen, Hilden, Germany). Real-time quantitative polymerase chain reaction (PCR) was performed using a Light Cyclr 480 II (Roche Diagnostics, Mannheim, Germany) with the Light Cycle 480 Probes Master Reaction Mix (Roche). PCR reactions were performed with the TaqMan Gene Expression Assay (Applied Biosystems, Foster City, CA, USA). The levels of target gene mRNA transcripts relative to control glyceraldehyde 3-phosphate dehydrogenase (GAPDH) were determined by 2–ΔΔCt. PCR amplifications were performed at 95°C for 15 seconds and subjected to 55 cycles of 95°C for 10 seconds, 60°C for 30 seconds, and 72°C for 1 second. The following TaqMan probes were used: Col1α1, SNAI1, SNAI2, and GAPDH (Table 2). Semi-quantitative reverse transcription-PCR amplifications were performed for TIMP1 and TIMP2 at 94°C for 5 minutes and subjected to 23 to 36 cycles of 94°C for 30 seconds, 56°C to 60°C for 30 seconds, 72°C for 1 minute, and a final extension for 5 minutes at 72°C. The primer sequences were as follows (5′ → 3′): TIMP1, 5′–AGACCTACACTGTTGGCTGTGAG–3′ and 5′–GACTGGAAGCCCTTTTCAGAG–3′; TIMP2, 5′–ATGCACATCACCCTCTGTGA–3′ and 5′–CTCTGTGACCCAGTCCATCC–3′; GAPDH, 5′–GAGTCAACGGATTTGGTCGT–3′ and 5′–TTGATTTTGGAGGGATCTCG–3′.
Western blotting analysis
Equivalent amounts of protein lysates (10 μg/lane) were separated by 10% sodium dodecyl sulphate–polyacrylamide gel electrophoresis (SDS-PAGE) and subjected to Western blot analysis with primary antibodies for α-SMA (Sigma-Aldrich, A2547), p-p38 (Cell Signaling Technology, #9211), p38 (Cell Signaling Technology, #9212), p-Smad2 (Cell Signaling Technology, #8828), and Smad2 (Cell Signaling Technology, #8685). Antibodies to α-tubulin (Sigma-Aldrich, T5168) served as loading controls.
Statistical analysis
Unpaired t tests or repeated measures analysis of variance (ANOVA) were used for statistical analysis. A two-sided p < 0.05 was considered statistically significant. Data was analyzed using SPSS version 20.0 (IBM Co., Armonk, NY, USA).
Ethical statement
No animal or human studies were carried out by the authors.
RESULTS
The effects of BCAAs on the activation of LX-2 cells cultured in low-amino acid medium
We evaluated the effects of BCAAs on LX-2 cells cultured in low-amino acid medium (Fig. 1). The LX-2 cells activated by TGF-β1 (2.5 ng/mL) adopted a flattened morphology (Fig. 1A). Induction of SNAI1 and SNAI2 expression is mediated by the TGF-β1/Smad signaling pathway [8,9], and expression of α-SMA, Col1α1, TIMP1, and TIMP2 is increased in activated HSC. Following treatment with 20 mM BCAA, the increased mRNA expression of SNAI1 on activated LX-2 cells significantly decreased (p = 0.036). Expression of Col1α1 and TIMP2 at the mRNA level decreased with 20 mM BCAA (p = 0.056 for both Col1α1 and TIMP2) (Fig. 1B and 1C). Western blotting showed that α-SMA expression increased with TGF-β1 (2.5 ng/mL) and decreased with BCAA treatments at 5 mM, 10 mM, and 20 mM without statistical significance (p = 0.183 for 5 mM BCAA, p = 0.098 for 10 mM, and p = 0.136 for 20 mM compared to the level in activated LX-2 cells without BCAA) (Fig. 1C). Unpaired t tests were used to assess for statistical difference.
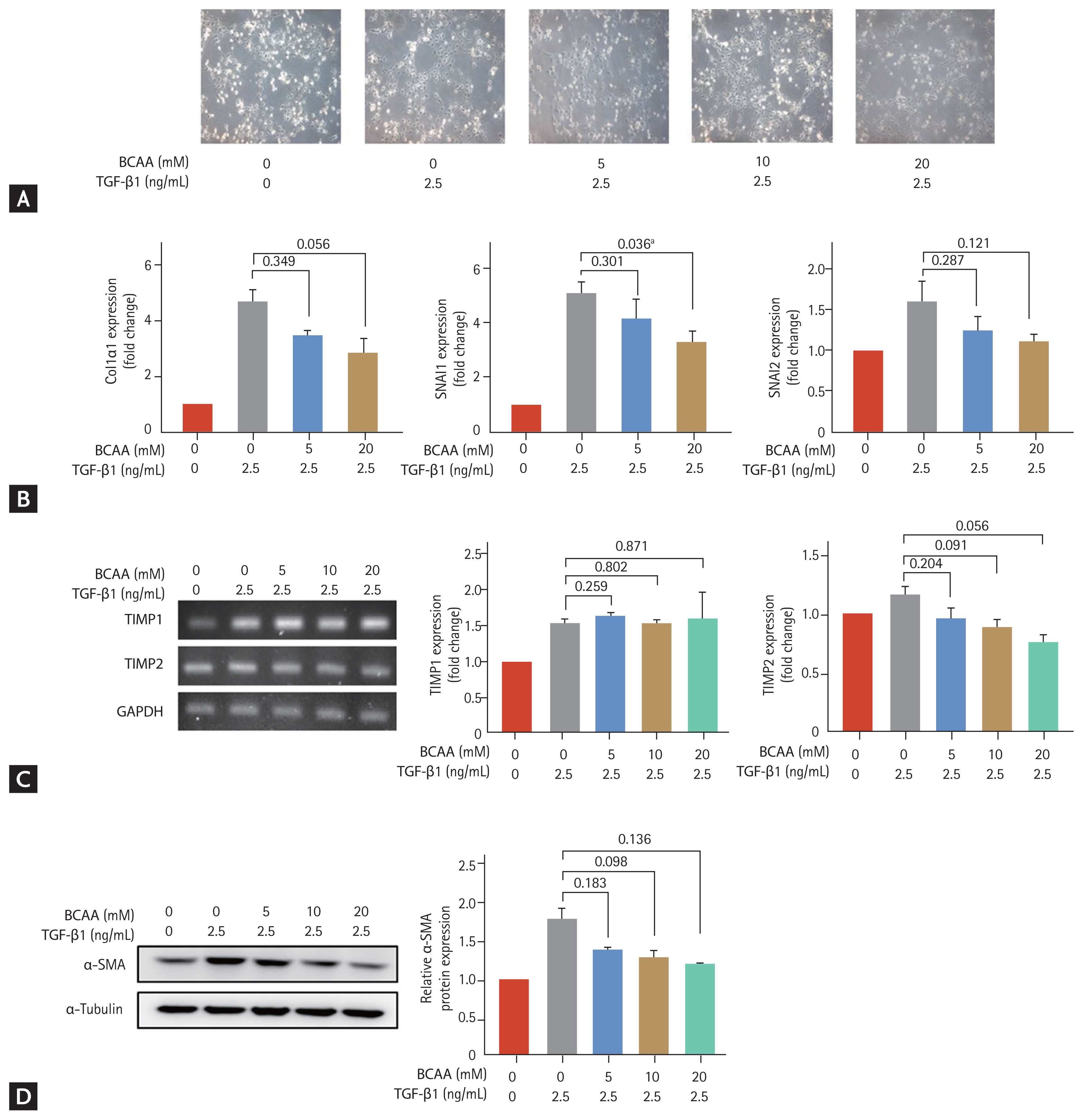
Effects of branched-chain amino acid (BCAA) treatment on activated LX-2 cells cultured in low-amino acid medium. Activated LX-2 cells were treated with 5, 10, and 20 mM of BCAA. (A) Morphological changes of LX-2 cells were observed under a microscope (100 × magnification). (B, C) Real-time quantitative polymerase chain reaction of collagen type I alpha 1 (Col1α1), snail family transcriptional repressor 1 (SNAI1), SNAI2, tissue inhibitor of metalloproteinase 1 (TIMP1), and TIMP2 was performed in LX-2 cells. (D) Western blotting of alpha smooth muscle actin (α-SMA) was performed in LX-2 cells. Bar graphs represent the mean ± standard error of mean (n = 3). Unpaired t tests were performed in LX-2 cells. TGF-β1, transforming growth factor β1; GAPDH, glyceraldehyde 3-phosphate dehydrogenase. ap < 0.05 compared to the level in activated LX-2 cells without BCAA.
The effect of each component of BCAA on the activation of LX-2 cells
We treated LX-2 cells that had been cultured in low-amino acid medium with valine, leucine and isoleucine at different concentrations (5, 10, and 20 mM) (Fig. 2). The increased expression of SNAI2 at the mRNA levels on activated LX-2 cells decreased with 20 mM leucine (p = 0.051) (Fig. 2A) and the increased expression of Col1α1 decreased with 20 mM valine (p = 0.083) (Fig. 2C). Protein expression of α-SMA in LX-2 cells increased with TGF-β1 (2.5 ng/mL) treatment and significantly decreased with 20 mM leucine treatment (p = 0.033 compared to the level in activated LX-2 cells without leucine) (Fig. 2B). Similarly, the increased protein expression of α-SMA with TGF-β1 (2.5 ng/mL) in LX-2 cells significantly decreased with 20 mM valine treatment (p = 0.024 compared to the level in activated LX-2 cells without valine) (Fig. 2D). There was no significant change in expression markers in activated LX-2 cells treated with isoleucine (Fig. 2E and 2F). Unpaired t tests were used to assess for statistical difference.
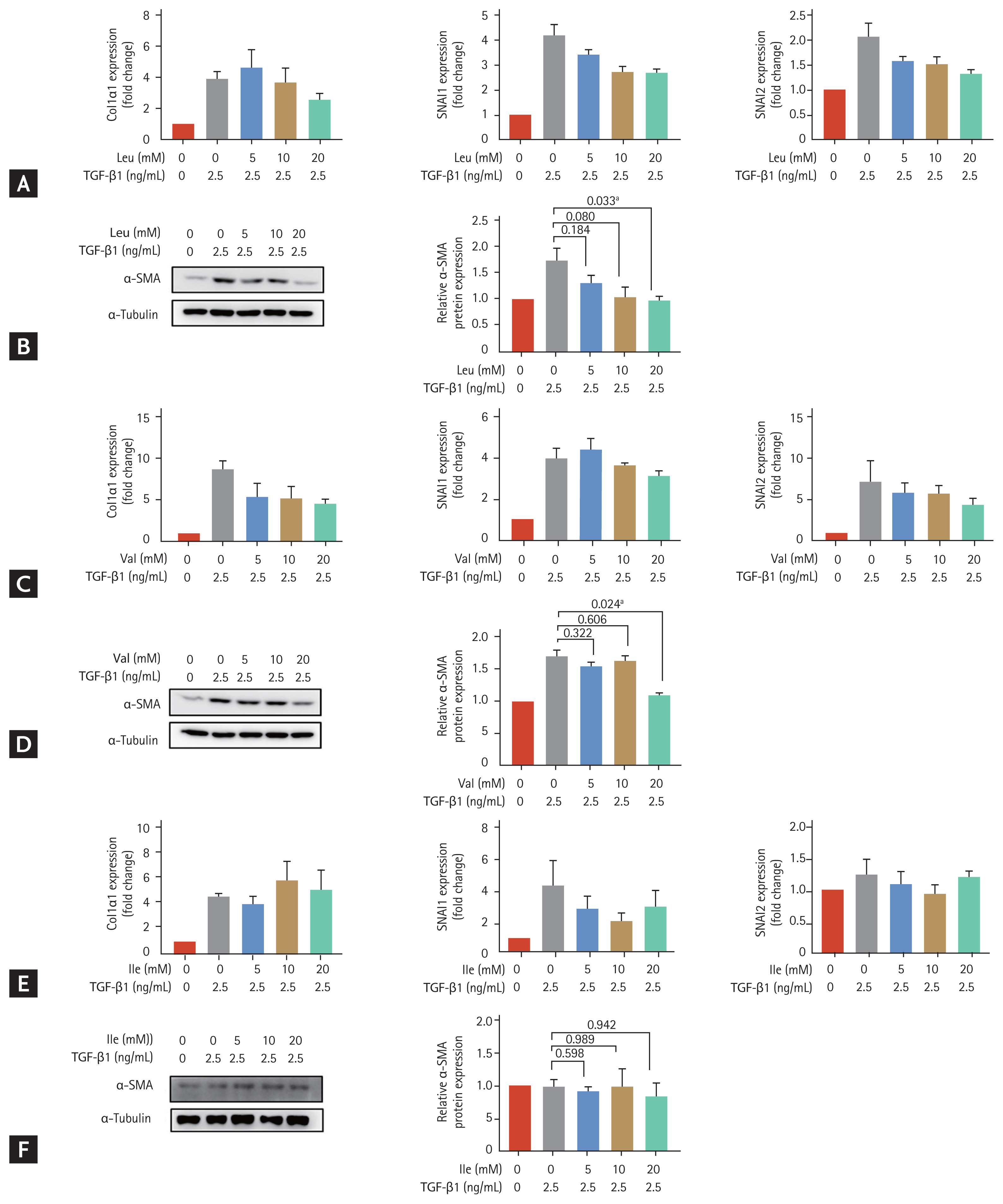
Effects of individual treatments with leucine, valine and isoleucine among the branched-chain amino acid (BCAA) components, on LX-2 cells activated by transforming growth factor β1 (TGF-β1) treatment. LX-2 cells were cultured in low-amino acid medium. Activated LX-2 cells were treated with 5, 10, and 20 mM of BCAA. Bar graphs represent the mean ± standard error of mean (n = 3). Unpaired t tests were performed. (A) Real-time quantitative polymerase chain reaction (PCR) of collagen type I alpha 1 (Col1α1), snail family transcriptional repressor 1 (SNAI1), and SNAI2 was performed in LX-2 cells treated with leucine. (B) Western blotting of alpha smooth muscle actin (α-SMA) was performed in LX-2 cells treated with leucine. (C) Real-time quantitative PCR of Col1α1, SNAI1, and SNAI2 was performed in LX-2 cells treated with valine. (D) Western blotting of α-SMA was performed in LX-2 cells treated with valine. (E) Real-time quantitative PCR of Col1α1, SNAI1, and SNAI2 was performed in LX-2 cells treated with isoleucine. (F) Western blotting of α-SMA was performed in LX-2 cells treated with isoleucine. GAPDH, glyceraldehyde 3-phosphate dehydrogenase; Ile, isoleucine; Leu, leucine; Val, valine. ap < 0.05 compared to the level in activated LX-2 cells without BCAA.
The expression of markers in the TGF-β signaling pathway
To investigate the role of BCAAs in the TGF-β signaling pathway of LX-2 cells, we analyzed the expression of molecular markers Smad2 and p38, which are involved in Smad and Smad-independent p38 mitogen-activated protein kinase (MAPK) pathways, respectively (Fig. 3). Western blotting showed that the increased phosphorylation of Smad2 in the Smad pathway by TGF-β1 (2.5 ng/mL) stimulation decreased after BCAA treatment in LX-2 cells (p = 0.032 with BCAAs of 5, 10, and 20 mM and p = 0.013 with BCAAs of 5 and 10 mM). The increased protein expression of p38 phosphorylation (p-p38) by TGF-β1 (2.5 ng/mL) in the p38 MAPK pathway also decreased by BCAA treatment (p = 0.008 with BCAA of 5, 10, and 20 mM, and p = 0.078 with BCAA of 5 and 10 mM). Repeated measures ANOVA was used to assess for statistical difference.
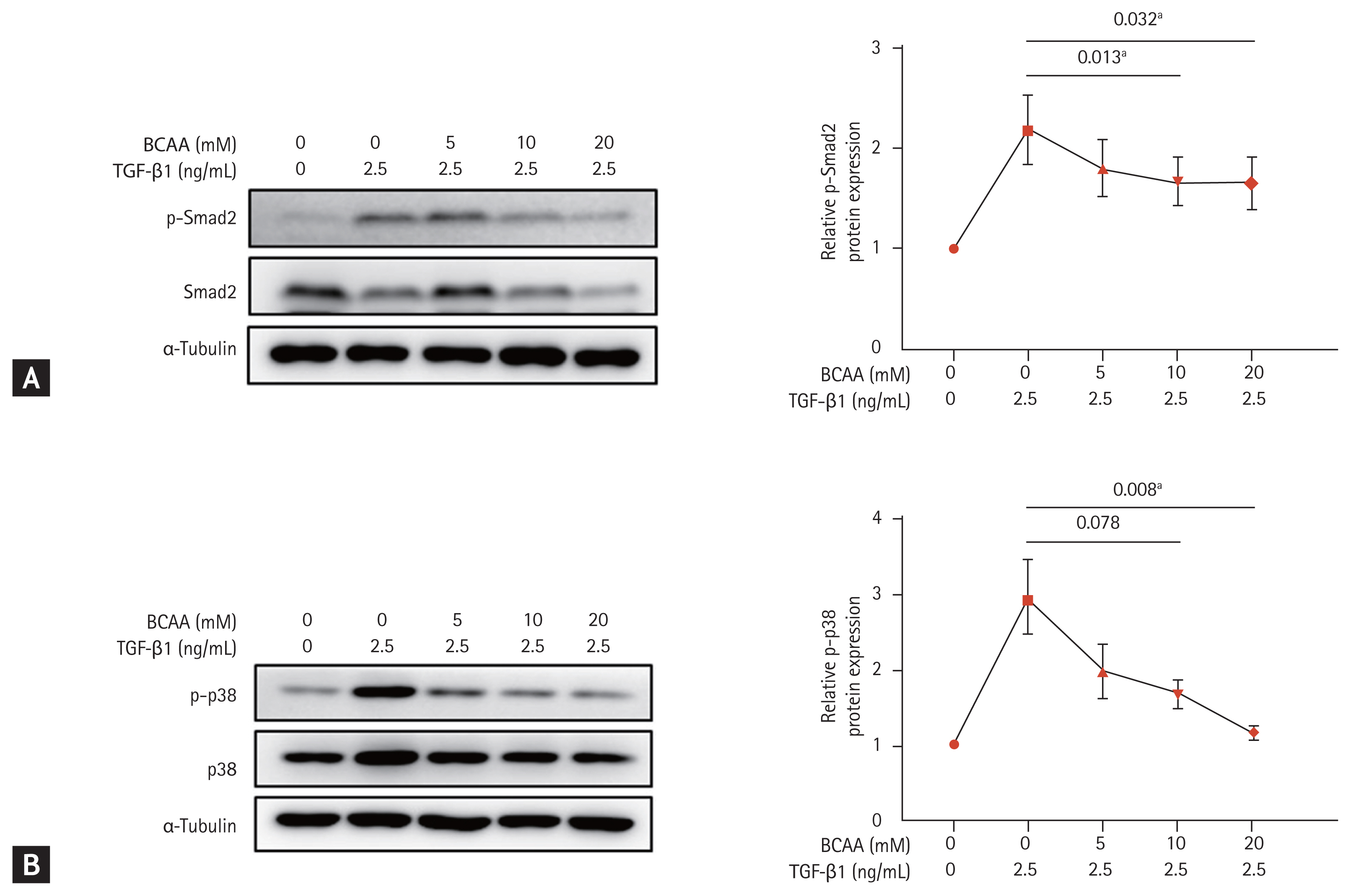
Change in expression of Smad2 and p38 phosphorylation (p-p38) in LX-2 cells activated by transforming growth factor β (TGF-β) treatment. LX-2 cells were cultured in low-amino acid medium. Activated LX-2 cells were treated with 5, 10, and 20 mM of branched-chain amino acid (BCAA). (A) Western blotting was performed against Smad2 phosphorylation (p-Smad2) in LX-2 cells. (B) Western blotting was performed against p-p38 in LX-2 cells. Means ± standard error of mean are shown (n = 3). Repeated measures analysis of variance (ANOVA) tests were performed. ap < 0.05 compared to the level in activated LX-2 cells without BCAA.
DISCUSSION
As several therapeutic approaches have been investigated to reverse cirrhosis, the role of HSCs, the major contributors to hepatic fibrosis, has been in the spotlight. Based on the recent results that BCAA supplementation can improve the prognosis of LC patients by inhibiting progression of cirrhosis, we focused on HSCs and studied whether BCAAs could reduce activation of HSCs. In this study, we identified that BCAAs exerted anti-fibrotic effects directly on HSCs. Leucine and valine, among the three components of BCAAs, showed inhibitory effects on activated LX-2 cells by TGF-β treatment. In addition, this study showed that BCAA treatment down-regulated expression of markers involved in Smad pathway and Smad-independent p38 MAPK pathway of TGF-β signaling in HSCs.
The positive effects of BCAA treatment in LC patients have been identified in various clinical aspects. In addition to the well-known effects of BCAAs on hepatic encephalopathy, recent studies demonstrated that BCAAs improved liver function in LC patients. A multicenter study with 2-year BCAA treatment in LC patients with Child-Turcotte-Pugh (CTP) class B showed that Model for End-Stage Liver Disease score, CTP score, and bilirubin level were significantly improved, and similar results were obtained from an Italian randomized controlled trial in which serum bilirubin level and CTP score were improved [10,11]. LC patients supplemented with long-term oral BCAA had a lower incidence of major cirrhosis-related events than control group in a prospective study [12]. Collectively, these results suggest that BCAA treatment improves hepatic reserve function and attenuates fibrosis process.
In this study, we tried to elucidate the detailed mechanism of BCAA effects on HSC activation in hepatic fibrosis. Expression markers such as α-SMA, Col1α1, SNAI1, and SNAI2, indicating LX-2 cell activation, significantly increased with TGF-β1 stimulation and decreased with BCAA treatment. We previously identified down-regulation of fibrosis markers including α-SMA, Col1α2, and Col3α1 at the protein and mRNA levels following BCAA treatment in rat liver tissue with induced fibrosis. Through this study, we demonstrated that BCAA directly inhibited activation of HSC. This is consistent with previous results that gene expression of TGF-β1 and Smad involved in the signaling pathway of HSC activation was significantly changed toward attenuating hepatic fibrosis by BCAA treatment, and the number of activated HSCs with expression of α-SMA clustered around fibrous septa decreased with BCAA treatment in rat liver tissue with CCl4-induced fibrosis [6]. Another study with LX-2 cells activated by TGF-β1 stimulation demonstrated that gene expression of markers related to the TGF-β1 signaling pathway, such as TGFβR1, p-Smad3, p-Smad3L, and α-SMA, significantly decreased with BCAA treatment [13].
The downstream pathway of TGF-β signaling to induce fibrosis is complex. TGF-β directly acts on HSCs and binds to TGF-β receptor 2, which subsequently activates TGF-β receptor 1 (TGFR1). This activated receptor phosphorylates Smad2 and Smad3 proteins, and phosphorylated Smad2/3 forms a complex with Smad4 to regulate transcription of ECM genes, such as collagens, plasminogen activator inhibitor1, and fibronectin in the nucleus [3,14]. In this study, we showed that expression of phosphorylated Smad2 increased with TGF-β1 treatment and significantly decreased with BCAA treatment in LX-2 cells, suggesting that the inhibitory effect of BCAAs acts on the stage before Smad2 is phosphorylated.
Aside from this canonical Smad pathway, activated TGFR1 induces other signal transducers, such as the Jun N-terminal kinases and p38 MAPK pathway, the phosphatidylinositol 3-kinase/protein kinase B (PI3K/Akt) pathway, and Rho family GTPases, collectively referred to as Smad-independent pathways [15–17]. In this study, increased expression of p-p38 by TGF-β stimulation was suppressed by BCAA treatment in LX-2 cells, indicating that BCAA could inhibit HSC activation through both Smad and Smad-independent p38 MAPK signaling pathways. Decreased expression of p-p38 by BCAA treatment was demonstrated in our previous study and was associated with suppression of tumor angiogenesis [7]. Activation of the p38 MAPK signaling pathway is associated with regulation of gene expression of transcription factors, cell surface receptors and cytokines, and the epithelial–mesenchymal transition process in hepatocytes [18,19]. Thus, it is speculated that the effect of BCAAs through inhibition of the p38 MAPK pathway is not limited to attenuating hepatic fibrosis by inhibiting HSC activation.
BCAAs is well-known activator for mammalian target of rapamycin complex 1 (mTORC1), and among the components of BCAAs, leucine, in particular, is known to be closely related to mTOR activation. It was suggested that the effect of BCAAs in TGF-β signaling pathway of HSC was through activation of mTORC1 [13,20,21]. In a previous study, leucine administration reduced the expression of phosphorylated TGFR1 and Smad2/3-positive nuclei compared to control groups in muscle tissue of rats, suggesting the effect of leucine on fibrosis attenuation [22]. In this study, although the effect of leucine was not remarkable, we identified that leucine and valine, except for isoleucine, reduced LX-2 cell activation.
This study has some limitations. We focused on investigating the mechanism of BCAA effect on HSC activation and used the human HSC cell line LX-2, widely used in culture-based studies for hepatic fibrosis. However, it is not enough to explain the effect of BCAA on HSC based only on the results from LX-2 cells. Second, this study identified decreased expression of phosphorylation of Smad2 proteins and p38 after BCAA treatment in the TGF-β signaling pathway in HSC, but we did not identify expression of other genes involved in different stages of Smad and Smad-independent pathways. Because of this, it was not possible to determine the specific steps that are inhibited by BCAAs in the TGF-β signaling pathway. Further study is needed to elucidate the precise mechanism of BCAAs in the process of hepatic fibrosis, which will also advance future research into development of anti-fibrotic therapies.
In conclusion, this study demonstrated that BCAA treatment could be beneficial for reducing hepatic fibrosis by direct action on HSCs to affect both Smad and Smad-independent p38 MAPK signaling pathways in TGF-β signaling in HSC. Among the BCAA components, leucine and valine showed an effect of anti-fibrosis. Through the findings of this study, we expect BCAAs to have a role in development of new anti-fibrosis therapies.
KEY MESSAGE
1. Branched-chain amino acid (BCAA) treatment inhibited transforming growth factor β (TGF-β)-induced activation of LX-2 cells.
2. Among the BCAA components, leucine and valine inhibited activated LX-2 cells.
3. BCAA treatment reduced Smad2 and p38 phosphorylation, which are involved in the downstream pathways of TGF-β1 signaling.
Notes
Conflict of interest
This study was supported by research grants from Hanmi Pharmaceutical Co., Ltd.
Acknowledgments
This research was supported by a grant of the Korea Health Technology R&D Project through the Korea Health Industry Development Institute (KHIDI), funded by the Ministry of Health & Welfare, Republic of Korea (grant number: HI17C1050).