Fundamental role of dendritic cells in inducing Th2 responses
Article information
Abstract
A mysterious puzzle in immunology is how the immune system decides what types of immune response to initiate against various stimuli. Although much is known about control of T helper 1 (Th1) and Th17 responses, the mechanisms that initiate Th2 responses remain obscure. Antigen-presenting cells, particularly dendritic cells (DCs), are mandatory for the induction of a Th cell response. Numerous studies have documented the organizing role of DCs in this process. The present review summarizes the fundamental roles of DCs in inducing Th2 responses.
INTRODUCTION
The immune system protects the host from pathogens, allergens, and chemicals that enter through mucosal surfaces. Diverse responses against these stimuli induce different types of immune response by various T helper (Th) cells. CD4+ Th cells comprise a diverse category of immune cells, including Th1, Th2, Th17, and T regulatory cells. These different types of Th cell have distinct cytokines and transcription factor profiles with distinct immune responses. For example, CD4+ Th1 responses are induced by intracellular bacteria and viruses, resulting in secretion of interferon γ (IFN-γ) and expression of signal transducer and activator of transcription (STAT)-4 [1-3]. On the other hand, Th2 cells produce cytokines such as interleukin (IL)-4, IL-5, IL-13, and IL-10 and express transcription factors, such as GATA binding protein-3, STAT-5, and STAT-6 [3-5].
Advances in immunology have revealed a fundamental role of the innate immune system in sensing pathogens and tuning the quality of Th responses. Th1 cells attack intracellular pathogens with cell-mediated immune responses, whereas Th2 cells protect against extracellular pathogens predominantly by helping with humoral responses. When the Th1 and Th2 cell responses are overreactive, the Th1 pathway induces organ-specific autoimmune diseases, such as arthritis and type 1 diabetes, whereas the Th2 pathway can predispose a host to systemic autoimmune diseases, such as allergy and atopic dermatitis [6,7].
Differentiation of Th cells into Th1 or Th2 cells is under the control of antigen-presenting cells (APCs), mostly dendritic cells (DCs). When DCs are exposed to intracellular pathogens, they move to lymph nodes and secrete IL-12, resulting in differentiation of naïve T-cells into Th1 cells. Those Th1 cells release IFN-γ, which restimulates the DCs to produce IL-12, resulting in differentiation of naïve T-cells into Th1 through an autocrine loop. Like that of Th1 cells, maturation of Th2 cells is initiated by IL-6 released from DCs, and these Th2 cells produce IL-4 to generate more Th2 cells through an autocrine loop to naive T-cells. Several cell types produce cytokines to mature Th2 cells and induce Th2 immune responses. Diverse pattern recognition receptors (PRRs) are involved, and multiple signaling pathways are elicited (Fig. 1) [8]. However, the fundamental roles of DCs in the Th2 immune responses associated with these factors are not apparent. Thus, in this report we summarize previous studies of DC-related Th2 immune responses and present a new signaling pathway, the cAMP/protein kinase A (cAMP/PKA) signaling pathway, as a target for Th2 immune disease.
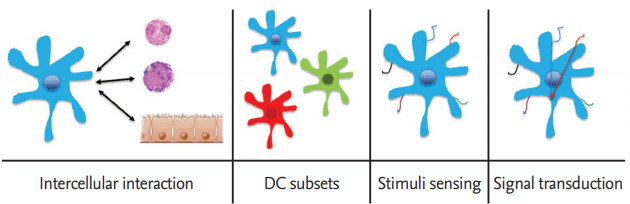
Levels that dendritic cells induce Th2 responses. For the induction of Th2 immune responses dendritic cells (DCs) require several steps in different levels of organization. Intercellular interaction between DC and other cells and specific DC subsets cause Th2 responses in upstream level of DC. In downstream level of DC, stimuli sensing and signal transduction of DCs are mandatory to induce Th2 responses.
INTERCELLULAR INTERACTIONS OF DENDRITIC CELLS IN TH2 IMMUNE RESPONSES
A microbe entering the body can activate various immune cells, such as natural killer cells, basophils, mast cells, regulatory T-cells, and diverse structural cells, such as tissue epithelial cells and stromal cells. Upon activation by microbes, DCs orchestrate the complex action of these cell types to initiate and regulate the Th2 immune response.
Among various immune cells, basophils initiate IL-4 production during the memory T-dependent response [9]. Furthermore, DCs or basophils alone are insufficient to induce the Th2 immune response in mice challenged by ovalbumin (OVA) plus papain, but cooperation between these two cell types allows DCs to induce T-cell proliferation and basophils to produce IL-4 for the Th2 immune response [10]. Therefore, the role of interaction between DCs and basophils in the induction of Th2 immune responses has been reviewed extensively [11-13]. However, the antigen-presenting role of basophils in Th2 immune responses described in these reviews is controversial because DCs are traditionally the major and sufficient APCs in Th2 immunity. Although basophils produce Th2 cytokines and are associated with induction of the Th2 immune response, whether they act as primary APCs in Th2 immunity is controversial. Taken together, data suggest that basophils behave as accessory cells to support DCs in inducing the Th2 immune response, in which DCs play a critical role as APCs and basophils produce Th2 cytokines, such as IL-4.
Innate lymphoid cells (ILCs) also orchestrate an efficient Th2 immune response in cooperation with tissueresident DCs. ILCs are classified as groups 1, 2 (ILC2), and 3, which generate antigen-specific adaptive immune responses. In particular, ILC2s are a potent source of Th2 cytokines that promote Th2 immune responses [14]. ILC2s react to helminth infection in the intestine and allergens in the airways by producing Th2 cytokines, such as IL-5 and IL-13 [15,16]. In recent reports, ILC2-derived IL-13 stimulated lung DCs to migrate into draining lymph nodes, where they induced naïve T-cells to differentiate into Th2 cells and produce the chemokine CCL17 (C-C motif chemokine ligand 17), which attracts CCR4+ (C-C motif chemokine receptor 4+) memory Th2 cells [17,18]. These results suggest that DCs stimulated by ILC2s at the epithelial barrier are critical in Th2 immune responses.
In addition to immune cells, epithelial cells have been studied as major players in Th2 immune responses that influence DC functions, although the epithelium was initially regarded as a physical barrier [19]. DCs are always covered by epithelium, which causes the DC sentinel function and activation of DCs in the lungs [20]. The epithelial junction is dysregulated in cases of allergic inflammation, and these epithelial cells are susceptible to Th2 cytokines [21]. Epithelial cells also produce thymic stromal lymphopoietin (TSLP) during allergic inflammation, which subsequently activates CD11c+ DCs to induce Th2 immune responses [22].
DENDRITIC CELL SUBSETS OF TH2 IMMUNE RESPONSES
As APCs, DCs mediate immunity with several discrete cell subtypes, differentiated from lymphoid or myeloid precursor DCs. These different DC subtypes have a common antigen-presenting capacity to T-cells and promote cell-cycle progression. However, they activate Tcells differently, resulting in various Th responses [23]. For example, mouse CD11c+CD8α– DCs from the spleen induce a Th2 response, whereas CD8α+ DCs lead to Th1 differentiation [24]. In another murine experimental set, the lymphoid-related DC subset (CD11c+CD8α+ DEC205+) induced Th1 cytokines, whereas the myeloidrelated DC subset (CD11c+CD8α–DEC205–) induced Th2 cytokines [25]. After OVA pulsing, DCs in the rat respiratory tract stimulated a Th2-dependent OVA-specific immunoglobulin (Ig) G1 response and mouse myeloid DCs induced Th2-dependent airway eosinophilia [26,27]. Mouse myeloid and plasmacytoid precursor DCs cultured from bone marrow precursors and ex vivo splenic DC subsets induced the development of Th1 or Th2 effector cells, depending on the antigen dose. In general, high antigen doses induce Th1 cell development, whereas low antigen doses induce Th2 cell development [28]. CD301b+ dermal DCs or bone marrow dendritic cells (BMDCs) drive the Th2 immune response in OT-II transgenic CD4+ T-cells [29,30].
In humans, monocyte-derived DCs induce the Th immune response differentially, depending on the ratio of DCs to T-cells. At a low ratio (1:300), mature DCs induce the transformation of naïve T-cells into Th2 effector cells, whereas a high DC/T-cell ratio (1:4) favors mixed Th1/Th2 cell development [31]. Human monocyte-derived DCs induce the Th1 response, whereas CD11c–CD1a– plasmacytoid DCs favor the Th2 immune response [32,33]. In terms of tissues other than blood, epidermal CD207+ Langerhans cells preferentially induce CD4+ T-cells to secrete Th2 cell cytokines [34].
STIMULUS SENSING AND PROCESSING OF DENDRITIC CELLS IN TH2 IMMUNE RESPONSES
Activated DCs sense a diverse array of pathogens and allergens by PRRs, such as Toll-like receptors (TLRs), C-type lectin-like receptors (CLRs), RIG-I-like receptors, and Nod-like receptors, which are expressed in the surface and intracellular areas of DCs. Triggering of these PRRs activates DCs, leading to antigen-specific activation of Th cells [35,36]. The means by which microbial stimuli signal through PRRs to induce Th1 immune responses are well understood, but our knowledge of the receptors that induce Th2 immune responses remains limited.
Among the PRRs on DCs, TLRs are the most studied. Various TLRs recognize microbial stimuli, such as lipopolysaccharide (LPS), lipoteichoic acids of Gram-positive bacteria and bacterial lipoproteins, and flagellin, and detect microbial nucleic acids, such as double-stranded RNA, single-stranded RNA, and CpG DNA. Although most TLR-eliciting signals induce Th1 responses, certain TLR ligands induce Th2 responses. Porphyromonas gingivalis LPS activates mouse DCs to produce Th2 cytokines through TLR4, whereas Escherichia coli LPS induces a Th1-like response [37]. The diacylated lipopeptide enhances production of Th2-type IgG1 antibodies in TLR2(+/+) mice, but not in TLR2(–/–) mice [38]. Stimulation of TLR2 with a synthetic TLR2 ligand elicits Th2 immune responses through extracellular regulated kinase (ERK) signaling in murine DCs [39]. In human DCs, TLR2 agonists yield a Th2 immune response, whereas triggering of TLR4 and TLR5 with E. coli LPS stimulates a Th1 response [40]. Activation of mouse DCs with a TLR2 ligand results in the induction of Th2 cytokines, such as IL-13, and promotes asthma in a mouse experimental model [41]. However, combined TLR2 and TLR4 activation by different antigens primes human DCs to induce Th1/Th2 immune responses [42]. Stimulation of human DCs by staphylococcal enterotoxin B through TLR2 drives naïve CD4 T-cells to develop a Th2 immune response [43]. Eosinophil-derived neurotoxin activates myeloid DCs by triggering the TLR2-myeloid differentiation factor 88 signaling pathway, which enhances an OVA-specific Th2 immune response [44]. In addition, TLR4 is necessary to induce Th2 responses to low-level LPS exposure in mouse DCs [45].
CLRs sense the carbohydrate regions of several pathogens and prime DCs to stimulate Th immune responses [46]. Dectins on mouse BMDCs and splenic DCs produce Th1 and Th17 cell responses, whereas DC-specific intercellular adhesion molecule-3–grabbing nonintegrin (DC-SIGN) induces a Th2 immune response on gastric DCs [47-49]. In addition, a ligand of DC-SIGN primes human DCs to induce the Th2 immune response [50].
Although PRR triggering of DCs is the main way to organize Th2 immune responses, other factors, such as the enzymes in allergens or alarmins from damaged tissue, can trigger Th2 immunity in the absence of PRR signaling [51]. IL-33 activates murine BMDCs to promote the Th2 immune response in allergic airway inflammation [52,53]. IL-25 also enhances the Th2 immune response of human TSLP-activated DCs [54].
Dendritic cell signal transduction in Th2 immune responses
Little is known about the signaling networks that stimulate DCs to induce a Th2 immune response. In contrast to LPS, which triggers TLR4 and induces DCs to initiate Th1 immune responses, the TLR2 ligand Pam3cys stimulates the duration and magnitude of the ERK mitogen-activated protein kinase in DCs and programs DCs to stimulate Th2 cell-biased responses [40]. These TLR2 ligands also elicit less IL-12p70 and more IL-10, consistent with other reports that Erk diminishes the induction of IL-12 and enhances IL-10 induction [55]. Furthermore, DCs from c-fos–/– mice promote IL-12 production and negatively regulate IL-10 [39]. Taken together, these results indicate that the Erk-Fos signaling pathway is an important regulator of IL-12 and IL-10 production in DCs and promotes the Th2 immune response.
Another signaling pathway that induces DCs to initiate the Th2 immune response is nuclear factor-κB (NF-κB). NF-κB is activated by TSLP and drives DCs to produce OX40L and induce the Th2 immune response [56]. Th2 cytokines are not expressed in DCs of NF-κB1–/– mice following injection of bacterial antigens [57]. Mouse BMDCs, stimulated by a helminth glycan, induce transient NF-κB translocation and activity in the nucleus and a Th2 immune response [58].
In addition to the above-described signaling pathways, knockout (KO) of Gnas, the gene that encodes Gαs in mouse CD11c+ cells, and the subsequent decrease of cAMP in mouse DCs were recently reported to provoke the Th2 immune response with an allergic phenotype, whereas increased cAMP has been found to induce Th17 immunity (Fig. 2) [59,60]. In this study, adoptive transfer of BMDCs from the Gnas KO mice induced Th2 immune responses, such as increased IL-4 and elevated IgE, and a PKA-selective cAMP agonist eliminated the Th2 phenotype in these mice. These results indicate that the Th2-biased effects of low cAMP concentration in DCs are regulated via PKA signaling and that cAMP/PKA signaling is an attractive target for the development of DC-directed therapy for Th2 immune diseases.
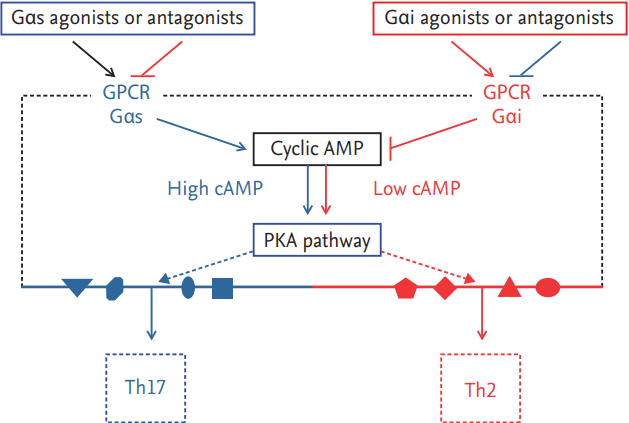
Novel signaling networks that provoke dendritic cell (DC) to induce Th2 or Th17 immunity. Decreased cyclic AMP (cAMP) concentration in dendritic cell provoke Th2 immunity via G-protein-coupled receptor (GPCR)/cAMP/ protein kinase A (PKA) signaling whereas increased cAMP concentration induce Th17 immunity through the same pathway. Gαs, stimulatory Gα subunit; Gαi, inhibitory Gα subunit.
Further study of the role of the DC-related signaling pathway in Th2 immune responses is required to broaden the range of intracellular signaling pathways, some of which have not been anticipated previously.
CONCLUSIONS
DCs interact with multiple types of immune and structural cells, show distinct subsets, bind to specific receptors according to the pathogen or allergen, and transfer stimuli through diverse signaling pathways to elicit Th2 immune responses. Although DC-related Th2 immune responses are too complex to identify a single main target factor and many aspects have not been revealed, newly discovered areas, such as cAMP/PKA signaling, illuminate the possibility of regulating Th2 immunity in the near future.
Notes
No potential conflict of interest relevant to this article was reported.
Acknowledgements
This research was supported by the Basic Science Research Program through the National Research Foundation of Korea funded by the Ministry of Science and Technology and the Ministry of Science, ICT & Future Planning (2014R1A1A1002131, NRF-2016R1E1A2020731, 2017R1A2B2003575) and the Korea Health Technology R&D Project (HI17C0387) through the Korea Health Industry Development Institute (KHIDI) by the Ministry of Health and Welfare. This research was also supported by a Korea University grant and a grant of Korea University Medical Center and Korea University Anam Hospital, Seoul, Republic of Korea (O1700021).