Induction of Macrophage Migration Inhibitory Factor in ConA-Stimulated Rheumatoid Arthritis Synovial Fibroblasts through the P38 MAP Kinase-Dependent Signaling Pathway
Article information
Abstract
Background/Aims
This study was undertaken to identify the intracellular signaling pathway involved in induction of macrophage migration inhibitory factor (MIF) in human rheumatoid arthritis (RA) synovial fibroblasts.
Methods
Human RA synovial fibroblasts were treated with concanavalin A (ConA), various cytokines, and inhibitors of signal transduction molecules. The production of MIF by synovial fibroblasts was measured in culture supernatants by ELISA. The expression of MIF mRNA was determined using reverse transcriptase polymerase chain reaction (RT-PCR) and real-time PCR. Phosphorylation of p38 mitogen-activated protein (MAP) kinase in synovial fibroblasts was confirmed using Western blotting. The expression of MIF and p38 MAP kinase in RA synovium was determined using dual immunohistochemistry.
Results
The production of MIF by RA synovial fibroblasts increased in a dose-dependent manner after ConA stimulation. MIF was also induced by interferon-γ, CD40 ligand, interleukin-15, interleukin-1β, tumor necrosis factor-α, and transforming growth factor-β. The production of MIF by RA synovial fibroblasts was significantly reduced after inhibition of p38 MAP kinase. The expression of MIF and p38 MAP kinase was upregulated in the RA synovium compared with the osteoarthritis synovium.
Conclusions
These results suggest that MIF production was induced through a p38 MAP-kinase-dependent pathway in RA synovial fibroblasts.
INTRODUCTION
Many cytokines play a central role in the formation of complex networks and cross-regulation to induce, augment, and regulate inflammatory cells in the pathogenesis of rheumatoid arthritis (RA) [1]. Inhibitors of various cytokines are used as effective therapeutic tools for severe RA. However, biological therapy, such as tumor necrosis factor (TNF)-α- or interleukin (IL)-1β-neutralizing agents have some limitations because blocking one cytokine gives incomplete control of pathogenesis in diseases that involve complex cytokine networks [1,2]. Therefore, combination therapy that blocks two cytokines or a cytokine and another signal transduction molecule is needed.
Concanavalin A (ConA) is a potential multi-receptor crosslinker for T-cell receptor and other cell-surface receptors. It is the most extensively investigated member of the lectin family of plant proteins and displays high affinity for terminal -D-mannosyl and -D-glucosyl residues [3]. ConA exhibits cell agglutinating and mitogenic activities and induces apoptosis [4]. ConA is a tetravalent lectin and is known to trigger augmented secretion and proteolytic activation of matrix metalloproteinases (MMPs) in fibroblasts; hence, it is widely used for the study of MMP secretion [5,6].
Macrophage migration inhibitory factor (MIF) is an important inflammatory cytokine in RA. MIF activates macrophages to induce the release of pro-inflammatory cytokines such as TNF-α, IL-1, and IL-6 and to promote interferon (IFN)-γ-induced production of nitric oxide [7,8]. MIF activates RA fibroblast-like synoviocytes (FLSs) to produce cyclooxygenase 2 (COX-2), MMP-1, and MMP-3, which contribute to tissue destruction [9,10]. MIF also upregulates MMP-13 mRNA [11], suppresses p53-mediated events in the inflamed synovium, and inhibits apoptosis of damaged cells [12,13]. The imbalance between the proliferation and apoptosis in the synovial cells results in tissue expansion in the RA synovium. Our previous study has shown that MIF controls angiogenesis in RA synovial fibroblasts by producing vascular endothelial growth factor (VEGF) and IL-8 and by inducing endothelial tube formation [14]. The presence of MIF reflects clinical disease activity in RA patients and might be useful as a clinical disease marker [14,15].
Although activated T cells are the main source, MIF is also expressed abundantly by FLSs and macrophages in the RA synovium, and FLS-derived MIF upregulates the release of TNF-α and IL-1β, suggesting that MIF acts as an upstream member of the network of cytokines that are operative in RA [7,14,16]. Recent data suggest that MIF regulates RA synovial hyperplasia by acting directly and indirectly via TNF-α and IL-1β. In addition, the effects of MIF on FLS activation and proliferation are dependent on extracellular signal-regulated kinase (ERK) and mitogen-activated protein (MAP) kinase, but are independent of nuclear factor-κB (NF-κB) [16,17]. Recombinant MIF activates RA FLSs to produce COX-2 and IL-6 via the p38 MAP kinase pathway [18]. Although the intracellular mechanism responsible for the effects of MIF on target cells has been investigated, the intracellular mechanism that underlies the activation of MIF in target cells such as RA synovial fibroblasts is unknown. We identified the signal transduction pathway involved in MIF induction after the stimulation of FLSs.
METHODS
Isolation and culture of FLSs
Synoviocytes were isolated by enzymatic digestion of synovial tissues obtained from patients with RA and osteoarthritis (OA) who were undergoing total joint replacement surgery. The tissues were minced into 2 to 3-mm pieces and treated for 4 hours with 4 mg/mL of type I collagenase (Worthington Biochemical, Freehold, NJ, USA) in Dulbecco's Modified Eagle's Medium (DMEM) at 37℃ in 5% CO2. Dissociated cells were then centrifuged at 500 g, resuspended in DMEM supplemented with 10% fetal bovine serum (FBS), 2 mM L-glutamine, 100 U/mL penicillin, and 100 µg/mL streptomycin, and plated in 75-cm2 flasks. After overnight culture, the nonadherent cells were removed, and the adherent cells were cultivated in DMEM supplemented with 20% FBS. The cultures were kept at 37℃ in 5% CO2, and the medium was replaced every 3 days. When the cells approached confluence, they were passed after 1:3 dilution with fresh medium. Synoviocytes from passages 4 to 8 were used in each experiment. The cells were morphologically homogeneous and exhibited the appearance of synovial fibroblasts, with typical bipolar configuration under inverse microscopy. The purity of the cells (1 × 104) was tested by flow cytometry using phycoerythrin-conjugated anti-CD14 and fluorescein-isothiocyanate-conjugated anti-CD3 or anti-Thy-1 (CD90) monoclonal antibodies (all from Pharmingen). At passage 4, most cells (> 95%) expressed the surface markers for fibroblasts (Thy-1), whereas 3.5% of cells were CD14+, and < 1% of cells were CD3+.
Measurement of MIF concentration in culture supernatants
MIF concentration was measured in culture supernatants by sandwich ELISA, as described previously [19]. Ninety-six-well plates were coated with 100 µL per well of 0.4 µg/mL goat anti-human MIF (R&D Systems, Minneapolis, MN, USA) buffered with 50 mM sodium bicarbonate (pH 9.6). After overnight incubation at 4℃, the plates were blocked with 1% bovine serum albumin (BSA) in PBS for 2 hours at room temperature. The human recombinant MIF (R&D Systems) or culture supernatant was added to the wells and then incubated for 2 hours at room temperature. Plates were incubated with 0.2 µg/mL of biotinylated goat anti-human MIF (R&D Systems) at room temperature for 2 hours. Peroxidase-labeled ExtrAvidin (Sigma Chemical Co., St. Louis, MO, USA), diluted 1:2,000, was added, and the plates were incubated at room temperature for 2 hours. A color reaction was induced by the addition of tetramethylbenzidine/H2O2 substrate solution and was stopped 30 minutes later by the addition of 1 M phosphoric acid. An automated microplate reader was used to measure the optical density at a wavelength of 450 nm. Between steps, plates were washed four times with PBS containing 0.05% Tween 20. Human recombinant MIF, diluted in culture medium over a concentration range of 10 to 2,000 pg/mL, was used as a calibration standard.
Measurement of MIF mRNA expression using reverse transcriptase polymerase chain reaction (RT-PCR)
mRNA was extracted from FLSs using RNAzol B according to the manufacturer's instructions (Biotex Laboratories, Houston, TX, USA). Two micrograms of total mRNA was reverse transcribed at 42℃ using the Superscript™ Revere Transcription System (Gibco BRL, Grand Island, NY, USA). PCR amplification of cDNA aliquots was performed by adding 2.5 mM dNTPs, 2.5 U Taq DNA polymerase (Takara, Shiga, Japan), and 0.25 µM of sense and antisense primers. The reaction was performed in PCR buffer (1.5 mM MgCl2, 50 mM KCl, 10 mM Tris HCl, pH 8.3) in a total volume of 25 µL. The primers used were MIF, 5'-GAACCGCTCCTACAGCAAGCT-3' (forward) and 5'-GCGAAGGTGGAGTTGT
TCCA-3' (reverse), and GAPDH, 5'-CGATGCTGGGCGTGAGTAC-3' (forward) and 5'-GTTCAGTCCAGGGATGACC-3' (reverse), which yielded PCR products of 381 and 300 bp, respectively. Reactions were processed in a DNA thermal cycler (PerkinElmer Cetus, Norwalk, CT, USA) through cycles of 30-second denaturation at 94℃, 1 minute annealing at 55℃ for GAPDH and at 56℃ for MIF, followed by 30-second elongation at 72℃. PCR rounds were repeated for 25 cycles for GAPDH and 35 cycles for MIF, which were determined to fall within the exponential phase of amplification for each molecule. The level of mRNA expression was presented as the ratio of MIF PCR product to GAPDH product.
Real-time PCR with SYBR Green
mRNA was extracted using RNAzol B (BioTex Laboratories) according to the manufacturer's instructions. Reverse transcription of 2 µg total mRNA was conducted at 42℃ using the Superscript Reverse Transcription System (Takara). PCR amplification of cDNA aliquots was performed by adding 2.5 mM dNTPs and 2.5 U Taq DNA polymerase (Takara), and human MIF was amplified using the sense primer 5'-CCGGACAGGGTCTACATCAACTATTAC-3' and the anti-sense primer 5'-TAGGCGAAGGTGGAGTTGTTCC- 3' in a LightCycler™ (Roche Diagnostics, Mannheim, Germany). The relative expression levels were calculated by normalizing the MIF levels to the endogenously expressed housekeeping gene β-actin. Melting curve analysis was performed immediately after the amplifi-cation protocol under the following conditions: 0 second (hold time) at 95℃, 15 seconds at 65℃, and 0 second (hold time) at 95℃. The temperature change rate was 20℃/sec except in the final step, when it was 0.1℃/sec. The crossing point (Cp) was defined as the maximum of the second derivative from the fluorescence curve.
Analysis of MAP kinase activation
The activation of ERK was antagonized with a specific inhibitor of MAP kinase/ERK kinase kinase (MEKK), PD98059 (Calbiochem, San Diego, CA, USA), and the specific p38 MAP kinase inhibitor was SB203580 (Calbiochem). The activation of NF-κB, activator protein 1, c-Jun N-terminal kinase (JNK), and Akt was antagonized with pyrollidine dithiocarbamate (PDTC; Sigma), curcumin (Sigma), JNK inhibitor (Calbiochem), and phosphoinositide 3-kinase (PI3K) inhibitor (Calbiochem), respectively. The phosphorylation of p38 MAP kinase was assessed using Western blotting with monoclonal antibodies specific for the phosphorylated (activated) form of p38 MAP kinase. RA synovial fibroblasts were stretched in the absence or presence of the pathway inhibitors indicated above for 1 hour. At various times, cells were scraped into lysis buffer, and the protein content was measured using the BioRad DC protein assay (BioRad Laboratories, Hercules, CA, USA). Equal amounts of protein were resolved by SDS-PAGE, transferred to a polyvinylidene fluoride membrane, and blotted with antibodies to the phosphorylated and nonphosphorylated forms of p38. A rabbit peroxidase-conjugated anti-rabbit antibody was used as the secondary antibody, and the binding was revealed by chemiluminescence reagents (ECL; Amersham Pharmacia Biotech, Piscataway, NJ, USA) according to the manufacturer's instructions.
Immunohistochemistry of RA synovium
Immunohistochemical staining for MIF and p38 MAP kinase was performed on sections of synovium. The synovial samples were obtained from RA patients, fixed in 4% paraformaldehyde solution overnight at 4℃, dehydrated with alcohol, washed, embedded in paraffin, and sectioned into 7-µm-thick slices. The sections were depleted of endogenous peroxidase activity by adding methanolic H2O2 and then blocked with normal serum for 30 minutes. After overnight incubation at 4℃ with polyclonal anti-human MIF and anti-p38 antibodies (Santa Cruz Biotechnology, Santa Cruz, CA, USA), the samples were incubated with the secondary antibody, biotinylated anti-rabbit IgG or biotinylated anti-goat IgG, for 20 minutes, and incubated with streptavidin-peroxidase complex (Vector, Peterborough, UK) for 1 hour, followed by incubation with 3,3'-diaminobenzidine (Dako, Glostrup, Denmark) for 5 minutes. The sections were counterstained with hematoxylin. Samples were photographed with an Olympus photomicroscope (Olympus, Tokyo, Japan).
Dual immunohistochemistry
Dual immunohistochemical labeling (MIF and p38) was performed using the DakoCytomation EnVision Doublestain Kit (code K1395; Dako North America, Carpinteria, CA, USA) according to the manufacturer's instructions. The synovial tissue was incubated with the first primary antibody (anti-MIF; R&D Systems) and polymer method, and the final color product was developed using aminoethyl carbazole (Dako). The second primary antibody (anti-p38; Santa Cruz Biotechnology) was placed on the sections at room temperature for 1 hour, followed by a standard immunohistochemical alkaline phosphatase method, and the color reaction was developed with fast blue. No counterstain was used, and the sections were mounted in an aqueous mounting medium. Samples were photographed with an Olympus photomicroscope.
Statistical analysis
The results were expressed as the mean ± SEM. Statistical analysis was performed using Student's t test and Wilcoxon signed-rank test. p < 0.05 was regarded as significant.
RESULTS
ConA-induced MIF production in RA synovial fibroblasts
To evaluate activated MIF production in RA synovial fibroblasts, we incubated RA synovial fibroblasts with various concentrations of ConA (0, 1, 5, or 10 µg/mL) for 24 hours, and the MIF concentration was measured in the culture supernatant using sandwich ELISA. Stimulation of synovial fibroblasts by ConA increased the production of MIF in a dose-dependent manner (Fig. 1A). To measure MIF mRNA expression, RA synovial fibroblasts were stimulated with ConA for 12 hours, and the MIF mRNA level was measured using semi-quantitative RT-PCR. GAPDH mRNA was used as the internal control for even loading. The pattern of mRNA expression was similar to that of MIF production measured by ELISA (Fig. 1B).
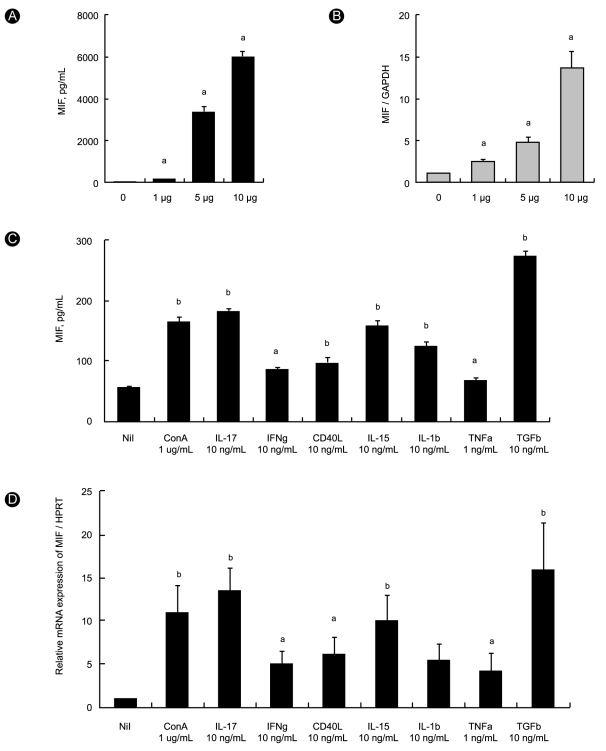
Effects of concanavalin A (ConA) and cytokines on production of migration inhibitory factor (MIF) in rheumatoid arthritis (RA) synovial fibroblasts. (A) RA synovial fibroblasts were treated with ConA (1 to 10 µg/mL) for 24 hours, and MIF concentration was measured in culture supernatants by sandwich ELISA. (B) RA synovial fibroblasts were treated with ConA for 12 hours, and MIF mRNA level was analyzed using RT-PCR. GAPDH mRNA was used as the internal control for even loading. Bars show the mean ± SEM of five separate experiments. Production of MIF by the synovial fibroblasts increased in a dose-dependent manner. (C) RA synovial fibroblasts were incubated with interferon (IFN)-γ, CD40L, interleukin (IL)-15, IL-1β, tumor necrosis factor (TNF)-α, or transforming growth factor (TGF)-β, and the concentration of MIF in the culture supernatant was measured using sandwich ELISA. (D) Expression of MIF mRNA was determined using real-time PCR. Data shown here represent synovial fibroblasts from five RA patients.
Effect of cytokines on the production of MIF in RA synovial fibroblasts
The effect of various inflammatory cytokines on MIF production was determined. RA synovial fibroblasts were incubated with IL-17 (10 ng/mL), IFN-γ (10 ng/mL), CD40 ligand (CD40L, 10 ng/mL), IL-15 (10 ng/mL), IL-1β (10 ng/mL), TNF-α (1 ng/mL), or transforming growth factor-β (TGF-β, 10 ng/mL) for 48 hours, and the concentration of MIF in the culture supernatant and the MIF mRNA level were measured using ELISA and real-time PCR, respectively. Inflammatory cytokines IL-17, IL-15, TNF-α, IL-1β, and IFN-γ or costimulatory molecule CD40L, which are involved in the pathogenesis of RA, increased production of MIF (Fig. 1C) and expression of MIF mRNA (Fig. 1D). Angiogenic cytokine TGF-β in RA synovium also increased MIF production (p < 0.01) and mRNA expression (p < 0.01).
Effects of signal inhibitors on the production of MIF in RA synovial fibroblasts
To determine which signal transduction pathway is involved in the induction of MIF, we used 10 nM LY294002, which antagonizes the activation of the PI3 kinase-Akt pathway, 10 nM SB203580, which antagonizes the activation of p38 MAP kinase, SP600125, which antagonizes the activation of JNK, and 300 µM PDTC, which antagonizes the activation of NF-κB. RA synovial fibroblasts were preincubated for 1 hour with the antagonist and then stimulated with 10 µg/mL of ConA for 24 hours. The production of MIF was measured in culture supernatants using the sandwich ELISA, and the expression of MIF mRNA was measured using RT-PCR in the synovial fibroblasts. MIF production decreased significantly after antagonism of p38 MAP kinase (p < 0.005), PI3K/Akt, and NF-κB (p < 0.05, Fig. 2A). The expression of MIF mRNA decreased significantly after antagonism of p38 MAP kinase (p < 0.05, Fig. 2B). These results show that MIF production in RA synovial fibroblasts is regulated mainly via p38 MAP kinase.
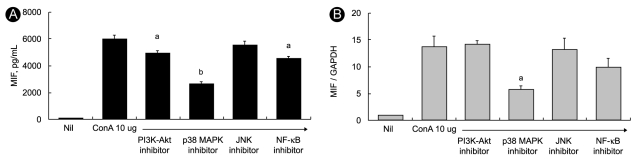
Effects of inhibition of intracellular signal molecules on the induction of migration inhibitory factor (MIF) in rheumatoid arthritis (RA) synovial fibroblasts. RA synovial fibroblasts were treated with inhibitors of signal molecules for 1 hour and then stimulated with concanavalin A (ConA; 10 µg/mL) for 24 hours. (A) Concentration of MIF was measured in culture supernatant using sandwich ELISA. Bars show the mean ± SEM of three separate experiments. (B) Expression of MIF mRNA was determined using RT-PCR. GAPDH mRNA was used as the internal control. MIF production and mRNA expression decreased significantly after inhibition of p38 mitogen-activated protein (MAP) kinase. In contrast, inhibition of phosphoinositide 3-kinase (PI3K)-Akt, nuclear factor-κB (NF-κB), and c-Jun N-terminal kinase (JNK) activity had no effect on the expression of MIF mRNA.
Activation of p38 MAP kinase requires phosphorylation, therefore, we investigated the phosphorylation of p38 MAP kinase after ConA stimulation in RA synovial fibroblasts. RA and OA synovial fibroblasts were cultured with stimulation by 10 µg/mL of ConA for 1 hour. Cell lysates were analyzed for p38 MAP kinase activation by Western blotting of total and Tyr182-phosphorylated p38 MAP kinase (phospho-p38 MAP kinase) using specific antibodies. The phospho-p38 MAP kinase level was normalized to that of p38 MAP kinase and β-actin in the same sample and compared at each time. ConA induced phosphorylation of p38 MAP kinase in RA, but not in OA synovial fibroblasts (data not shown). However, the ConA-induced phosphorylation of p38 MAP kinase was reduced after treatment with SB203580 (Fig. 3A).
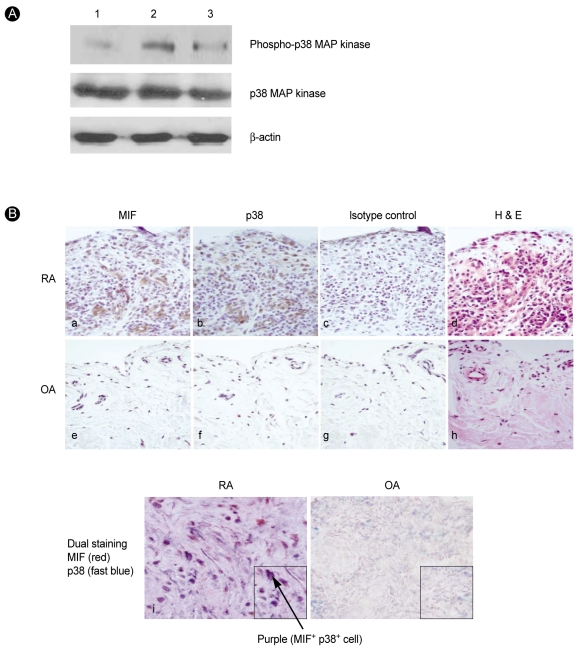
Phosphorylation of p38 mitogen-activated protein (MAP) kinase in rheumatoid arthritis (RA) synovial fibroblasts. (A) RA synovial fibroblasts were cultured with or without concanavalin A (ConA) stimulation (10 µg/mL) for 1 hour. Lysates were examined for p38 MAP kinase activation by Western blotting with p38 MAP kinase phosphospecific antibodies. Total p38 MAP-kinase-specific antibody was used to verify equal protein loading. Lane 1, RA synovial fibroblasts without ConA treatment; lane 2, RA synovial fibroblasts with ConA treatment; lane 3, treatment with ConA and p38 MAP kinase inhibitor SB203580 (10 nM). Data shown here represent one of three independent experiments. (B) Expression of migration inhibitory factor (MIF) and p38 MAP kinase increased in the RA synovium. Expression of MIF and p38 MAP kinase in the RA and osteoarthritis (OA) synovium was detected using immunohistochemical staining. All tissues were counterstained with hematoxylin (× 400). (a) Staining for MIF in RA synovium, (b) staining for p38 MAP kinase in RA synovium, (c) isotype control in RA synovium, (d) hematoxylin and eosin staining in RA synovium, (e) staining for MIF in OA synovium, (f) staining for p38 MAP kinase in OA synovium, (g) isotype control in OA synovium, (h) H & E staining in OA synovium, (i) dual staining for p38 MAP kinase (fast blue color) and MIF (red color) in RA synovium, and (j) in OA synovium.
Expression of MIF and P38 in RA synovium and synovial fibroblasts
We observed the constitutive expression of MIF in RA synovial tissues using immunohistochemical staining. In the synovial tissues, more intense staining of MIF and p38 MAP kinase was observed in the RA intima and subintima compared with the OA synovium (Fig. 3B). Some cells that were positively stained with MIF (red) also expressed p38 MAP kinase (fast blue), which revealed the existence of merged cells (purple) that co-expressed MIF and P38 (Fig 3B).
DISCUSSION
Since MIF was first identified in 1966, its influence on the pathogenesis of many diseases, including RA, has been investigated extensively [20,21]. Many studies have shown that MIF plays a pro-inflammatory role in many inflammatory diseases, such as sepsis [22-24], RA [7,15,25,26], delayed-type hypersensitivity [27], glomerulonephritis [28-30], and various tumors [31-34]. In the pathogenesis of RA, MIF regulates RA synovial hyperplasia directly and by its effects on TNF-α and IL-1β, and activation of T cells [8,35].
MIF is released from T cells, macrophages, fibroblast-like synoviocytes, and endothelial cells in patients with RA and has both paracrine and autocrine actions that stimulate the activation of these cells [8]. It is also overexpressed in serum, synovial fluid, cultured synovial fibroblasts, and synovial tissues of RA patients [7,15,36]. One study has shown that MIF immunostaining of RA synovium correlates with the disease activity of RA, as assessed by C-reactive protein (CRP) concentration, tenderness, and swollen joint count [15]. In our previous study, the synovial concentration of MIF was correlated with erythrocyte sedimentation rate, CRP concentration, and oral steroid dosage, and synovial MIF was upregulated in patients with radiographic bony erosions [14]. These data suggest that MIF plays an important role in RA pathogenesis and that its expression reflects the clinical state of disease.
Although the membrane receptor for MIF has not been extensively studied, the intracellular signal transduction pathways for MIF are partially understood. One experimental study has shown that MIF binds to the extracellular domain of CD74, a type II transmembrane protein with high affinity, and that CD74 is required for MIF-induced activation of intracellular signal transduction pathways and cell activation and proliferation [37]. The transcriptional co-activator, c-Jun activation domain-binding protein 1, has been identified as an intracellular receptor protein for MIF, and the ERK MAP kinase pathway as the MIF-mediated signaling pathway [17].
TNF-α, IL-1, and IL-6 induce activation of ERK, JNK, and p38 MAP kinase in cultured human synovial cells [38]. In contrast, IFN-γ, IL-4, IL-10, and TGF-β do not activate these signal pathway molecules [39]. P38 MAP kinase is activated in response to IL-1β and TNF-α in human synovial fibroblasts and is also involved in IL-6 and IL-8 synthesis [40,41]. The double phosphorylation and translocation from the cytosol into the nucleus of p38 MAP kinase result in the initiation of transcription by binding to regulatory sites on DNA. Thus, activation of pro-inflammatory cytokines such as TNF-α, IL-1, IL-6, and IL-8 plays a central role in the pathogenesis of RA. In addition, an inhibitor of the activation of p38 MAP kinase, SB 203580, blocks the production and action of IL-6 and IL-8 in synovial fibroblasts [41] and TNF-α and IL-1 in bone and cartilage [42,43]. The potent inhibitor of p38 MAP kinase, FR167653, prevented onset of arthritis in a prophylactic treatment model and suppressed the progression of joint destruction in a therapeutic treatment model [44].
As far as we are aware, no studies have examined the signal transduction pathway involved in the production of MIF. In the pathogenesis of RA, the p38 MAP kinase pathway has an important role in the regulation of proinflammatory cytokines, synthesis of MMPs, formation of osteoclasts, and activation of endothelial cells. As a result, p38 MAK kinase is involved in synovial inflammation, cartilage degradation, inflammatory bone loss, and angiogenesis [45]. In this study, we observed that MIF production decreased significantly after inhibition of p38 MAP kinase and partially after inhibition of Akt and NF-κB; however, inhibition of JNK had no effect on MIF production in RA synovial fibroblasts. These results suggest that the p38 MAP kinase pathway is a main pathway in the induction of MIF in RA.
MAP kinase signaling has been found at distinct sites in synovial tissue, and p38 MAP kinase activation has been observed in the synovial lining and endothelial cells. We also found that ConA induced phosphorylation of p38 MAP kinase in RA synovial fibroblasts, but not in OA synovial fibroblasts. MIF actives RA FLSs to express COX-2 and IL-6 via p38 MAP kinase, and MIF regulates glucocorticoid sensitivity in macrophages, also via p38 MAP kinase [18,46]. Taken together, previous data and our own suggest that inhibition of p38 MAP kinase is effective in regulating induction of MIF expression and MIF-induced gene activation. The expression of MIF and p38 MAP kinase was upregulated in the RA synovium. ConA stimulation induced MIF mainly through a p38 MAP-kinase-dependent pathway in RA synovial fibroblasts. The inhibition of p38 MAP kinase might be effective in regulating the induction of MIF expression and induced gene activation.
Acknowledgments
This work was supported by a grant (A092258-0911-1070100) and (A092258-0911-1030100) from the Korea Healthcare Technology R&D Project, Ministry for Health, Welfare and Family Affairs, Republic of Korea
Notes
No potential conflict of interest relevant to this article was reported.