2024 KSoLA consensus on secondary dyslipidemia
Article information
Abstract
Elevated blood cholesterol and triglyceride levels induced by secondary causes are frequently observed. The identification and appropriate handling of these causes are essential for secondary dyslipidemia treatment. Major secondary causes of hypercholesterolemia and hypertriglyceridemia include an unhealthy diet, diseases and metabolic conditions affecting lipid levels, and therapeutic side effects. It is imperative to correct secondary causes prior to initiating conventional lipid-lowering therapy. Guideline-based lipid therapy can then be administered based on the subsequent lipid levels.
INTRODUCTION
Pharmacological lipid-lowering is an essential measure for cardiovascular prevention in modern medicine [1,2]. Elevated blood cholesterol and triglyceride (TG) levels are commonly induced by secondary causes [3]. If secondary dyslipidemia is suspected, diagnosing and managing the causative factors are critical, as is lipid level monitoring. Therefore, screening for the influence of diet, disease, and drug-induced lipid changes may be an important procedure for clinicians. Furthermore, as many physicians may be insufficiently aware of the side effects of medications that they do not often prescribe [4], relevant supplemental knowledge can help differentiate secondary dyslipidemia.
Many guidelines on lipid-lowering therapy (LLT) address secondary dyslipidemia [5,6]. Although these guidelines describe the causative conditions and the significance of their screening and treatment, these are only briefly described. Nevertheless, dyslipidemia holds a solid position in contemporary clinical medicine [7,8] and has a variety of secondary causes. Therefore, the accumulation and summary of detailed data and a recommended approach for suspected cases are required. Consequently, we reached a consensus regarding secondary dyslipidemia. For practical use by clinicians, hypercholesterolemia and hypertriglyceridemia are presented separately. In addition, three major etiologies— diet, diseases, and drugs—were explained, and recommendations were made. Secondary causes of dyslipidemia are presented in Figure 1 according to their impact on lipoprotein synthesis and clearance. The therapeutic approach for these patients is suggested in Figure 2.
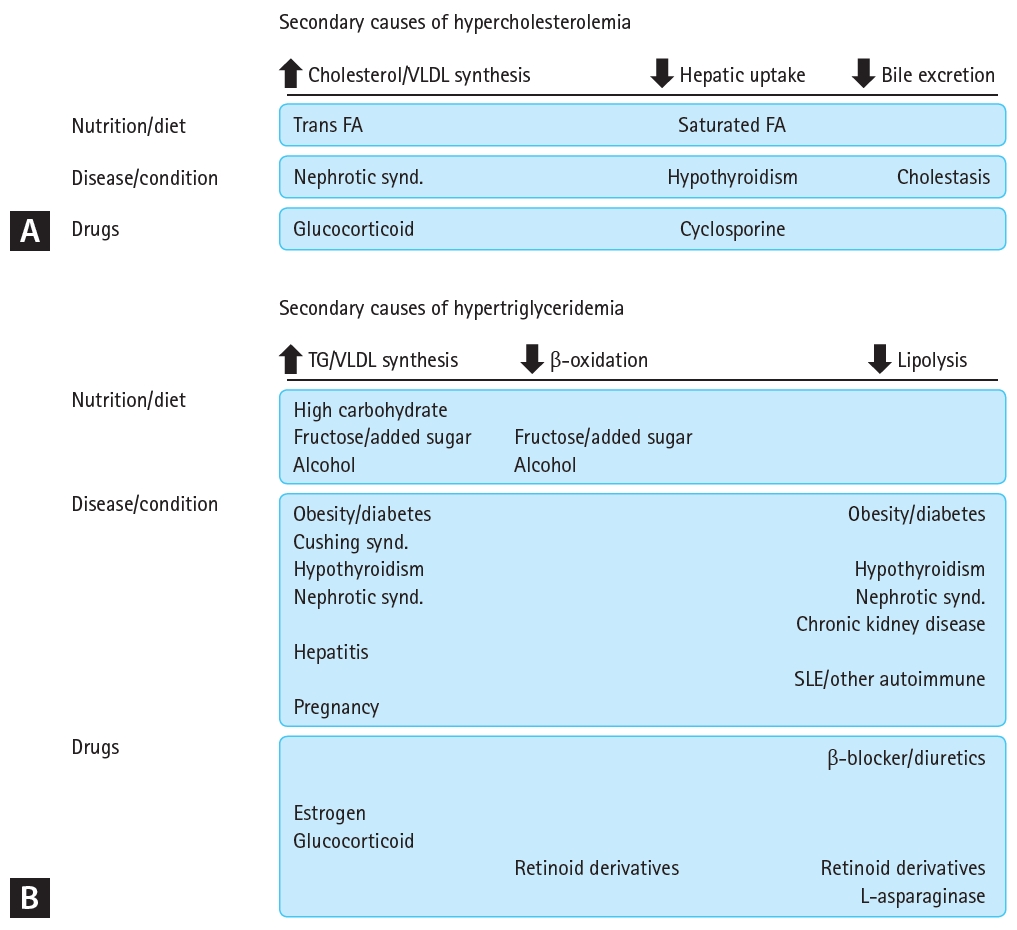
Secondary causes of hypercholesterolemia (A) and hypertriglyceridemia (B) based on their impact on lipoprotein synthesis and clearance. VLDL, very low-density lipoprotein; FA, fatty acid; TG, triglyceride; SLE, systemic lupus erythematosus.
NUTRIENT, FOOD, AND DIETARY PATTERN-ASSOCIATED HYPERCHOLESTEROLEMIA AND HYPERTRIGLYCERIDEMIA
A lifestyle that accelerates obesity, including excessive calorie intake and low physical activity, commonly worsens hypertriglyceridemia [9].
Nutrients
Excessive energy intake
Obesity, especially abdominal obesity, is a major cause of secondary dyslipidemia. Therefore, an appropriate energy intake is important. The timing of daily energy intake also affects total cholesterol and low-density lipoprotein-cholesterol (LDL-C) levels. A Taiwanese study found that shifting consumption of 100 kcal from nighttime to breakfast or lunch could reduce LDL-C by 1.5 mg/dL [10]. Cholesterol synthesis in humans increases in the evening and night, possibly due to the peak activity of 3-hydroxy-3-methyl-glutaryl-coenzyme A reductase.
Saturated fatty acids (FAs)
Saturated FAs are a key factor in elevating LDL-C levels. Saturated FAs are associated with dyslipidemia in individuals whose diet includes saturated FAs at ≥ 10% of the total energy intake [11]. Specifically, saturated FAs with 12–16 carbon atoms tend to increase LDL-C levels [12]. Palm oil, including palmitic acid, elevates LDL-C levels by 9 mg/dL compared with other vegetable oils [13]. The most plausible underlying mechanism is the effect of saturated FAs on LDL receptor (LDLR) activity [14]. Polyunsaturated FAs can upregulate the mRNA and protein expression of LDLR and downregulate FA synthase. Conversely, monounsaturated FAs decrease apoC3 and apoB100 synthesis, which are the underlying mechanisms for lipid lowering by unsaturated FAs [15].
Trans FAs
Trans FA consumption increases blood LDL-C and TG levels to a greater extent than isocaloric intake of saturated FAs [16]. In human cells, elaidic acid, the most common trans FA, increases the expression of enzymes involved in cholesterol and FA biosynthesis compared with oleic acid [17].
High carbohydrate
A diet high in carbohydrates raises blood TG levels and decreases high-density lipoprotein-cholesterol (HDL-C) levels. Increased insulin concentrations resulting from a high-carbohydrate diet stimulate fat synthesis by elevating malonyl-CoA, which contributes to the conversion of FAs from oxidative to TG synthesis [18]. A low-carbohydrate diet leads to an improvement in blood TG and HDL-C levels compared with a low-fat diet [19]. A low-carbohydrate diet decreases TG levels to a greater extent than a moderate-carbohydrate diet, whereas it increases LDL-C [20]. LDL-C levels increase as carbohydrate intake decreases [21]. These results indicate the potential risks and benefits of a low-carbohydrate diet and underscore carbohydrate replacement over carbohydrate restriction.
Fructose
Adding fructose to the diet to boost energy intake increases blood TG levels. Associations between fructose and simple sugars, increased lipogenesis, and very low-density lipoprotein (VLDL) production have also been highlighted. Consuming ≥ 100 g of fructose per day or added sugars ≥ 10% of the total energy intake is known to elevate blood TG levels [9]. Fructose has been shown to increase (postprandial) TG concentrations only in hypercaloric trials [22].
Food
Red meat
Excessive consumption of red meat, a major source of saturated FAs, is associated with the risk of dyslipidemia. In a Korean cohort study, a one serving (60 g) increase in red meat intake was associated with a higher risk of hypercholesterolemia in both men and women [23].
Added sugars and sugar-sweetened beverage (SSB)
According to data from the National Health and Nutrition Examination Survey in the United States, as the percentage calorie intake from added sugar increased, the risk of hypertriglyceridemia increased [24]. An analysis of the Framingham Offspring study showed that more than one serving of SSB per day caused a 1.52 times higher risk of TG elevation than less than one serving per day [25]. High sugar intake enhances fructose metabolism, which mostly occurs in the liver. Excessive fructose accumulation increases de novo lipogenesis and inhibits FA oxidation, which in turn increases lipid supply in the liver. Elevated lipid levels promote VLDL secretion [26].
Furthermore, although non-nutritive sweeteners are often used as alternatives to sugar, the World Health Organization (WHO) has announced that the long-term consumption of non-nutritive sweeteners may be associated with the risk of non-communicable diseases [27].
Ultra-processed food
Consuming ultra-processed foods, which mainly contain free sugars, trans FAs, and saturated FAs, is a risk factor for dyslipidemia. A study that analyzed the intake of ultra-processed foods according to the NOVA classification identified the followings. Individuals in the highest tertile had a 2.7 times higher risk of hypertriglyceridemia than those in the lowest tertile [28]. In a Korean cohort study, the increased consumption of processed meat was associated with a higher risk of hypercholesterolemia and hypertriglyceridemia [23].
Alcohol
Every 1 oz of alcohol intake in non-drinkers increases blood TG levels by 5–10% [29]. Excessive alcohol intake suppresses hepatic FA oxidation, thereby promoting hepatic TG synthesis and VLDL secretion [9,30]. In particular, TG elevation can be more severe in individuals with underlying hypertriglyceridemia [9].
Dietary patterns
Dietary habits characterized by an excessive intake of refined grains, saturated fats, and sodium have a negative impact on lipid metabolism. In 2023, the American Heart Association highlighted issues with the Northeast Asian diet, including the Korean diet, that increase cardiovascular risk: the absence of dietary fiber in refined grains, such as white rice, high saturated fat and dietary cholesterol from animal protein and organ meats, and high sodium intake [31]. According to cancer screening data from the Korean National Cancer Center, Western dietary patterns, characterized by a higher intake of bread, snacks, noodles, meat, pizza, hamburgers, sugar, oil, and fat, were associated with a higher risk of total cholesterol and LDL-C levels [32].
Recommendations
Consume an appropriate energy intake to maintain a healthy body weight
Appropriate energy intake to maintain healthy weight is required. Energy needs vary according to individual’s age, sex, size, and activity [33]. Weight loss reduces cholesterol and TG levels [34]. In excessive adiposity, a 5% decrease in body weight reduces LDL-C by 3–5% [35].
Any diet that results in weight reduction, regardless of nutrient composition, can decrease blood TG levels. A study comparing four diets with different nutritional contents found that all four reduced TG levels and weight to similar extents [36]. For overweight individuals with hypertriglyceridemia, a 5–10% weight reduction is reasonable and is expected to lower TG levels by 20% [9]. Nonnutritive sweeteners are not recommended for weight control or risk reduction of these diseases.
Reduce saturated FA intake to < 7% of total energy, and limit trans FA intake
The 2020 Dietary Reference Intakes for Koreans recommends an intake of saturated FA < 7% and trans FA < 1% of total energy [37]. A greater intake of monounsaturated FAs (nuts, olive oil, etc.) and marine omega-3 polyunsaturated FAs is recommended [9]. Replacing saturated fat with unsaturated fat lowers lipid levels and cardiovascular risk [38,39], and substituting with unsaturated FAs and carbohydrates lowers LDL-C levels by 3 mg/dL [40]. In addition, substituting red meat with high-quality plant protein sources such as legumes leads to a reduction in total cholesterol and LDL-C [41].
Consume foods high in dietary fiber to reach a daily intake > 25 g
A meta-analysis revealed that high dietary fiber intake reduces total cholesterol compared with low fiber intake, with 25–29 g/day showing health benefits [42]. Dietary fiber, especially water-soluble fiber, reduced intestinal fat absorption and increased hepatic LDLR with lower VLDL secretion, thereby lowering blood cholesterol levels in animals [43]. The WHO recommends consuming at least 400 g of fruits and vegetables per day to increase dietary fiber intake [44]. Similarly, the 2020 Dietary Reference Intakes for Koreans recommends dietary fiber ≥ 30 g/day and ≥ 20 g/day for adult men and women, respectively [37].
Promote the consumption of whole grains, fruits and vegetables, legumes, and fish while reducing red and processed meat
The WHO emphasizes the consumption of whole grains, fruits and vegetables, legumes, and nuts to prevent noncommunicable diseases [44]. These foods are also recommended by international guidelines for LDL-C management and cardiovascular health [45].
The benefits of carbohydrate replacement have been emphasized over those of carbohydrate restriction. To improve dietary principles and personalized nutritional recommendations for secondary dyslipidemia, referral to a dietitian nutritionist is recommended [46]. The optimal diet for those with elevated TG levels is fiber-rich and includes < 50–60% carbohydrates, mostly consisting of whole grains, fruits, and vegetables [9].
Limit alcohol consumption to one to two glasses per day, and refrain from drinking if possible
The European Society of Cardiology recommends reducing alcohol consumption to lower TG levels [5]. The dietary guidelines for Americans limit alcohol consumption to two cups per day for men and one cup per day for women [47]. Furthermore, they recommended that people who do not consume alcohol refrain from starting to drink [33], as alcohol can exacerbate TG elevation in individuals with baseline hypertriglyceridemia; complete abstinence is recommended for these individuals.
DISEASES AND METABOLIC CAUSES CONTRIBUTING TO SECONDARY HYPERCHOLESTEROLEMIA
Hypothyroidism
Hypothyroidism is commonly accompanied by increased plasma LDL-C levels and sometimes mild TG elevation. Thyroid hormone has opposing effects on cholesterol synthesis and clearance. It induces HMG-CoA reductase expression in the liver and activates sterol regulatory element-binding protein 2. This protein binds to sterol regulatory elements in the LDLR promoter and induces hepatic LDLR expression. In addition, thyroid hormone upregulates LDLR expression by binding to the thyroid response element of the LDLR gene [48]. Therefore, hyper-LDL cholesterolemia in this condition is mainly caused by reduced hepatic LDLR and delayed LDL clearance [30]. Furthermore, FA oxidation can decrease and VLDL production is increased in individuals with hypothyroidism. Recently, thyroid-stimulating hormone has also been reported to influence lipid metabolism. It possibly promotes cholesterol synthesis and inhibit LDL clearance. This hormone could also increase TG synthesis [48].
Liver disease and primary biliary cholangitis (PBC)
As the liver is the primary site of lipoprotein metabolism, liver diseases can influence blood lipid levels in diverse ways. Cholestasis is associated with hypercholesterolemia, as bile is a major route of cholesterol excretion and cholestasis blocks this pathway. In this condition, free cholesterol is bound to phospholipids and transported to the blood as a particle called lipoprotein X, which is characterized by a scarcity of apoprotein B [30,49].
PBC is an autoimmune liver disease. Its lipid profile is characterized by elevated levels of lipoprotein X and HDL, and low LDL-C levels. However, altered lipoprotein metabolism in PBC is not associated with a high cardiovascular risk [49].
Nephrotic syndrome
Dysregulation of lipid metabolism correlates with proteinuria in nephrotic syndrome, and blood cholesterol, TG, VLDL, intermediate-density lipoprotein, and lipoprotein (a) levels are elevated. These changes are primarily due to increased VLDL production. Although the underlying mechanisms remain unclear, protein overproduction associated with hypoalbuminemia is a potential cause [30]. Dyslipidemia likely contributes to the increased risk of atherosclerosis associated with this condition. Furthermore, dyslipidemia can cause renal injury and the progression of chronic kidney disease [50]. Although statins have shown cardiovascular benefits in patients with dialysis-independent chronic kidney disease, there are no data on statin treatment in nephrotic syndrome [50].
Recommendations
All patients with high LDL-C levels should be screened for hypothyroidism, particularly those in which the elevation is otherwise clinically unexplainable. Thyroid hormone replacement therapy usually improves hypercholesterolemia [30,51]. Laboratory screening tests for diseases causing secondary hypercholesterolemia or hypertriglyceridemia are presented in Table 1.
Physicians may be concerned about the hepatotoxicity of LLT in liver disease. Patients with liver disease may require LLT due to a combination of risk factors. In PBC, apoB levels may help determine who should start LLT. For patients needing such therapy, statins are the first-line agents, but caution should be exercised when administered to patients with decompensated cirrhosis [49].
DRUG-INDUCED SECONDARY HYPERCHOLESTEROLEMIA
Drugs inducing secondary hypercholesterolemia or hypertriglyceridemia are summarized in Table 2.
Cardiovascular drugs (e.g., thiazide and loop diuretics)
Thiazide diuretics increase LDL-C levels by 5–15% and these effects are known to be short-term. Loop diuretics have similar effects on lipid levels, whereas indapamide does not [52].
Steroid hormones (e.g., progestogens, androgenic anabolic steroids, and glucocorticoids)
Steroid hormones include estrogens, progestins, estrogen receptor modulators, and glucocorticoids. The original progestins, which are derivatives of testosterone, increase LDL-C levels. However, of the newer generations of progestins with varying androgenic effects, only dl-norgestrel increases LDL-C levels, whereas the others increase TG levels [53]. In contrast, combined estrogen/progestogen therapy decreases LDL-C levels. Oral contraceptives with second generation progestogens such as levonorgestrel mildly elevate LDL-C levels, whereas those with third generation progestogens, including desogestrel, do not. Danazol, a synthetic steroid prescribed for endometriosis, fibrocystic breast disease, and hereditary angioedema, increases LDL-C levels by 10–40%; however, these recover after discontinuation of the drug [52].
Androgenic anabolic steroids or drugs that increase their endogenous forms are sometimes used to enhance athletic performance or the sense of well-being. These drugs have been shown to increase LDL-C levels. These agents decrease lipoprotein lipase (LPL) activity and can reduce VLDL catabolism [53].
Glucocorticoids are used in various conditions. Prednisone increases LDL-C levels in a dose-dependent manner in patients who are post-transplant. In patients with systemic lupus erythematosus (SLE), prednisone increases LDL-C levels by up to 20%. Glucocorticoids demonstrate dose- and duration-dependent effects on lipid metabolism. In several studies, glucocorticoid use is accompanied by insulin resistance, which can confound the effects of steroids on lipid levels. The effect of glucocorticoids on post-heparin LPL activity varies [53]. In addition, glucocorticoids increase the hepatic production of VLDL by promoting rate-limiting enzyme activity [54].
Dermatologic drugs (e.g., isotretinoin)
Isotretinoin, a synthetic retinoid prescribed for acne vulgaris, mildly elevates LDL-C levels. An increased VLDL turnover induced by retinoids is a potential mechanism [53].
Immunosuppressants (e.g., cyclosporine and rapamycin)
Patients taking immunosuppressive drugs after transplantation may develop dyslipidemia. These agents can elevate LDL-C levels by 0–50%, with cyclosporine having the greatest increase. Moreover, the effects on lipid levels are greater in women. Immunosuppression can further increase small dense LDL particle levels. In addition, rapamycin has a prominent effect on cholesterol levels [52]. Although its mechanism of action on lipids remains unclear, cyclosporine can reduce LDL catabolism by inhibiting LDLR synthesis [55]. Furthermore, it also reduces bile acid synthesis [53].
Anti-infective protease inhibitors (e.g., ritonavir, indinavir, and nelfinavir)
These antiretroviral drugs administered against human immunodeficiency virus infection, many of which are currently less commonly used, increase LDL-C levels by 15–30% [52,56]. This effect develops after prolonged use (≥ 1 year) and is dose-responsive [57].
Anticonvulsants (e.g., carbamazepine, phenytoin, and phenobarbital)
These drugs are known to increase total cholesterol by 0–15%. Anticonvulsants, except for valproic acid, can induce enzymes in the hepatic microsomal cytochrome P pathway and compete with cholesterol metabolism via this route [52].
Others (e.g., sodium-glucose cotransporter-2 [SGLT2] inhibitors)
SGLT2 inhibitors, when used alone, minimally raise LDL-C levels by 2–4%. Increased synthesis and decreased catabolism underlie these changes. However, their effect on lipid levels is frequently altered when SGLT2 inhibitors are used in combination with statins and other diabetes medications in clinical practice [58].
Recommendations
Replacing drugs that induce hypercholesterolemia with alternative equivalents is desirable. For example, renin-angiotensin system blockers can be used as alternatives for patients with hypertension and dyslipidemia induced by cardiovascular agents. However, finding an appropriate alternative is often challenging. If a drug is necessary and difficult to replace, lipid levels must be monitored regularly. Reassessing the necessity of the causative drug and the risks and benefits of continuing dyslipidemia-inducing agents is essential. Guideline-based non-pharmacological and pharmacological LLT may be required with long-term use of causative agents. If a dyslipidemia-inducing drug is required for a short period, LLT is not necessary [52].
When using statins in post-transplant hypercholesterolemia, caution should be exercised regarding drug–drug interactions. In particular, a combination of cyclosporine and statins may increase the risk of myopathy, and statins that do not use the CYP3A4 pathway, such as fluvastatin and pravastatin, are preferred. Cyclosporine has been reported to elevate blood levels of ezetimibe, a second-line lipid-lowering agent [5].
Although research on dyslipidemia induced by protease inhibitors is limited, modifying the protease inhibitor regimen or initiating LLT are possible options. However, the risks and benefits of regimen changes and drug interactions between protease inhibitors and statins need to be carefully considered. Lovastatin and simvastatin are contraindicated in patients receiving protease inhibitors because they are metabolized by CYP3A4. Although atorvastatin is metabolized to a lesser extent by this pathway, the dose sometimes needs to be reduced. Fluvastatin, pravastatin, pitavastatin, and rosuvastatin can be used for these patients [56].
DISEASES AND METABOLIC CAUSES CONTRIBUTING TO SECONDARY HYPERTRIGLYCERIDEMIA
Obesity and uncontrolled diabetes
Among the medical conditions that cause TG elevation, obesity and poorly controlled diabetes are the most common. These conditions frequently result in elevated plasma TG, low HDL-C, variable LDL-C, and increased small density LDL levels. Large VLDL, increased TG in LDL and HDL, glycation of apoproteins, and high susceptibility of LDL to oxidation are qualitative differences in diabetes. Even if the blood LDL concentration is normal, its circulation time is longer in diabetes. VLDL production is increased, and its catabolism is decreased, whereas HDL catabolism is promoted. Postprandial hyperlipidemia is commonly observed [59]. Greater adipocyte mass and decreased insulin sensitivity of hormone-sensitive lipases result in excessive hepatic VLDL production from the overflow of free FA [9,30]. Furthermore, the increased insulin levels promote hepatic FA synthesis. In addition, insulin resistance reduces LPL activity and decreases the catabolism of chylomicrons and VLDL [9,30,60]. Insulin resistance and the subsequent decrease in LPL transcription and increase in LPL inhibitor levels may partly contribute to impaired lipolysis [30]. Furthermore, low clearance of TG-rich lipoproteins independent of LPL activity has been reported in type 2 diabetes. Moreover, insulin infusion promotes LPL expression in adipocytes [9].
Cushing syndrome
This condition commonly results in elevated TG levels and low HDL-C levels [30]. Excess glucocorticoids increase VLDL synthesis and cause hypertriglyceridemia.
Hypothyroidism
Hypothyroidism increases TG levels by affecting TG metabolism. Low thyroid hormone levels lead to increased VLDL TG production in the liver. LPL activity is downregulated, reducing the breakdown of TG-rich lipoproteins. In addition, impaired hepatic lipase activity contributes to the accumulation of these lipoproteins. Conversely, thyroid stimulating hormone promotes TG synthesis in adipocytes and hepatocytes [48].
Nephrotic syndrome
Lipid metabolism is altered in nephrotic syndrome. In particular, blood cholesterol, TG, and apoB-containing lipoprotein levels are elevated. By downregulating catabolizing enzymes such as LPL, hepatic lipase, and VLDL receptors, this disease can impair the clearance of VLDL and chylomicrons. Hypoalbuminemia increases hepatic protein synthesis, which is one of plausible underlying mechanisms [61].
Chronic kidney disease
This condition is often associated with mildly elevated TG levels. Lipolysis and clearance of remnant lipoproteins are decreased in affected patients. In these patients, the expression and activity of LPL are reduced. Furthermore, insulin resistance induced by parathyroid hormones and other conditions is a contributing factor. The clearance of VLDL particles is compromised in uremia because VLDL receptors are downregulated. Conversely, chronic kidney disease can increase the expression of enzymes involved in TG synthesis [62].
Liver disease
Hepatitis induced by infection, drugs, or alcohol commonly increases VLDL synthesis and causes mild-to-moderate TG elevation. In contrast, severe hepatic conditions such as liver failure can lead to dramatic decreases in blood cholesterol and TG levels [30].
Autoimmune diseases, including SLE
Patients with SLE have decreased LPL activity, and some produce anti-LPL antibodies [63]. Hyperchylomicronemia has also been reported in patients with a variety of other autoimmune diseases, such as polymyositis/dermatomyositis, rheumatoid arthritis, and Sjogren’s syndrome, and these patients were positive for anti-LPL antibodies [64]. However, some researchers have indicated that measuring anti-LPL antibodies is complicated. On the other hand, some affected patients have anti-GPIHBP1 antibodies [65]. Improving hypertriglyceridemia with steroid therapy can help to diagnose autoimmunity-associated hypertriglyceridemia.
Sepsis and critical illnesses
TG levels increase, whereas HDL-C and LDL-C levels decrease in these patients. Free FA levels increase, indicating enhanced tissue lipolysis, which is more prominent in patients in septic shock [66].
Pregnancy
Blood TG levels can increase up to 4-fold in the third trimester. However, they frequently remain <250 mg/dL, and are well-tolerated. An increase in estrogen and human placental lactogen levels during this period contributes to insulin resistance and TG elevation. Estrogen increases VLDL synthesis and impairs lipolysis; human placental lactogens also inhibit lipolysis [67].
Recommendations
The aim of TG lowering is to prevent acute pancreatitis and atherosclerotic cardiovascular disease. While chylomicron particles increase the risk of the former, smaller remnant particles may increase the risk of the latter [9]. Correcting secondary factors of hypertriglyceridemia is crucial before implementing other TG-lowering measures. For example, weight reduction through caloric restriction can reduce TG levels by approximately 10% [9]. Physical activity and exercise are known to reduce TG levels up to 30%. However, when managing individuals with secondary dyslipidemia, it is essential to personally target greatest contributor among secondary etiologies [46].
Anti-LPL antibodies may lead to elevated TG levels in patients with autoimmune diseases. However, measuring anti-LPL antibody levels is impossible in most clinical settings. This antibody is usually assayed by immunoblotting or enzyme-linked immunosorbent assay using purified LPL from post-heparin plasma. However, these methods have some technical limitations [63].
If TG levels are > 225 mg/dL during pregnancy, regular monitoring and lifestyle modifications are required. If TG levels are > 500 mg/dL despite implementing non-pharmacological measures, omega-3 FAs may be initiated [67].
DRUG-INDUCED SECONDARY HYPERTRIGLYCERIDEMIA
Some drugs cause mild changes in blood TG levels, whereas others cause severe changes (Table 2) [46].
Cardiovascular drugs (e.g., b-blockers, thiazide, and loop diuretics)
Mild-to-moderate TG elevation occurs after the administration of b-blockers, particularly nonselective ones (atenolol, propranolol, and metoprolol). In addition, thiazide and loop diuretics cause mild increases in TG levels [9,52]. Although the underlying mechanisms are unclear, b-blockers and diuretics without intrinsic sympathomimetic activity increase reflex a-adrenergic activity, which can inhibit LPL and increase TG levels. Diuretics have been associated with worsening insulin resistance that may contribute to lipid changes [53].
Steroid hormones (e.g., estrogen, oral contraceptives, tamoxifen, and glucocorticoids)
Unopposed estrogens used as oral hormone replacements may increase TG levels by 30–40% in a dose-dependent manner and are associated with increased VLDL production [9,52,53]. In contrast, the influence of transdermal estrogens without a first-pass effect is minimal. The effects of combined estrogen/progestogen therapy on TG levels are inconsistent. Oral contraceptives with second generation progestogens, including levonorgestrel, and third generation progestogens, such as desogestrel, increase TG levels by up to 50–75%. Although tamoxifen, a selective estrogen receptor modulator, can increase TG levels by 0–30%, severe elevations are uncommon [9,52]. The effects of tamoxifen appear to be related to lipase inhibition [53].
Post-transplant, prednisone increases TG levels, but not in a dose-dependent manner. In healthy individuals and patients with lupus, TG levels increase by up to 40% with glucocorticoid use [53]. This effect arises from increased VLDL production and the concomitant hyperinsulinemia [54].
Dermatologic drugs (e.g., isotretinoin and acitretin)
Variable degrees of TG elevation (35–144%) have been reported after the use of isotretinoin, a retinoid derivative. This results from the inhibition of FA oxidation and an increase in apoC3 [9]. Acitretin, used for severe psoriasis, can increase TG levels by up to 60% [52].
Immunosuppressants (e.g., cyclosporine and rapamycin)
These agents are commonly used after transplantation and may increase TG levels by 0–70% [52]. In general, the effect of cyclosporine on TG levels is less consistent than its effect on LDL-C levels.
Anti-infective agents-protease inhibitors (e.g., ritonavir, indinavir, and nelfinavir)
Ritonavir can elevate TG levels by 200–300%, whereas indinavir and nelfinavir cause an increase of 0–55% [52].
Anti-cancer drugs (e.g., L-asparaginase and cyclophosphamide)
L-asparaginase infrequently induces severe hypertriglyceridemia and is associated with LPL inhibition [9].
Antipsychotics (e.g., olanzapine and mirtazapine)
Second-generation antipsychotics are associated with mild-to-severe hypertriglyceridemia. Among these, clozapine and olanzapine are associated with a high risk of elevated TG levels [9]. Haloperidol causes a TG elevation of approximately 50%, although this effect is only seen in women [52].
Recommendations
Before administering drugs known to considerably increase TG levels, these should be measured both before initiating therapy and weeks later, which can be helpful for monitoring the effects of the causative agent. When very severe hypertriglyceridemia occurs, discontinuation and avoidance of the causative agents are required to prevent pancreatitis. In addition, hypertriglyceridemia is a contraindication for some drugs, including isotretinoin [52,68]. When treating patients with post-transplant hypertriglyceridemia, drug interactions between fibrate and cyclosporine must be considered [5].
CONCLUSIONS
Various causes including diet, diseases, and drugs can contribute to secondary elevation of blood cholesterol and TG. Screening and identification of potential causes are primary steps for secondary dyslipidemia. Managing secondary factors, especially focusing on the greatest contributor, needs to be preceded before additional treatment.
Acknowledgements
This paper is published in duplicate in the Korean Journal of Internal Medicine and the Journal of Lipid and Atherosclerosis.
Notes
CRedit authorship contributions
Hoyoun Won: methodology, writing - original draft, writing - review & editing; Jae Hyun Bae: methodology, writing - original draft, writing - review & editing; Hyunjung Lim: methodology, writing - original draft, writing - review & editing; Minji Kang: methodology, writing - original draft, writing - review & editing; Minjoo Kim: methodology, writing - original draft, writing - review & editing; Sang-Hak Lee: conceptualization, methodology, writing - original draft, writing - review & editing, visualization, project administration, funding acquisition
Conflicts of interest
The authors disclose no conflicts.
Funding
This work was supported by the Korean Society of Lipid and Atherosclerosis. The funders had no role in the study design, data collection and analysis, decision to publish, or preparation of the manuscript.