Clinical effects of non-pharmaceutical interventions for COVID-19 on other nationally notifiable infectious diseases in South Korea
Article information
Abstract
Background/Aims
This study aimed to assess the impact of non-pharmaceutical interventions (NPIs) implemented during the COVID-19 pandemic on nationally notifiable infectious diseases (NNIDs) in South Korea.
Methods
Long-term data on seven NNIDs from 2018 to 2021 were analyzed to identify trends and change points using a change point detection technique. The timings of the NPI implementations were compared to the identified change points to determine their association.
Results
Varicella, mumps, and scarlet fever showed a significant decrease in incidence following the implementation of NPIs during the COVID-19 pandemic. These diseases, which are primarily transmitted through respiratory droplets, demonstrated a clear response to NPIs. However, carbapenem-resistant Enterobacterales (CRE) showed an increasing trend unrelated to the timing of NPI implementation, suggesting the complex nature of controlling healthcare-associated infections. Hepatitis A, hepatitis C, and scrub typhus did not show significant changes associated with NPIs, likely due to their non-respiratory route of transmission.
Conclusions
NPIs effectively controlled NNIDs, particularly those transmitted through respiratory infections. However, the impact varied depending on the disease. Understanding the effectiveness and limitations of NPIs is crucial for developing comprehensive public health strategies during infectious disease outbreaks.
INTRODUCTION
The COVID-19 pandemic has highlighted the importance of non-pharmaceutical interventions (NPIs) in controlling the spread of infectious diseases. NPIs are interventions that do not involve the use of pharmaceuticals, such as vaccines and drugs, but instead focus on changing human behavior and social norms to reduce the transmission of pathogens. These interventions can range from simple measures like handwashing and wearing masks to more drastic measures like lockdowns and school closures.
NPIs have been widely used in the past to control infectious disease outbreaks. The Centers for Disease Control and Prevention in the US recommended the use of NPIs to suppress severe acute respiratory syndrome (SARS) during the 2003 outbreak (https://archive.cdc.gov/#/details?url=https://www.cdc.gov/sars/index.html) [1], while several other studies have also reported the positive effects of NPIs on respiratory tract infections [2,3]. Furthermore, NPIs implemented during the COVID-19 pandemic differed greatly from those administered during other disease outbreaks because they were strictly implemented at the national level. Most people complied with these strict regulations in the early days of COVID-19, meaning that the control measures were relatively effective. South Korea was one of the countries that initially implemented particularly stringent NPIs, and the spread of the disease was successfully controlled as a consequence [4,5]. Therefore, South Korea can be an important case study for determining the effects of NPIs when they are initiated in the early stages of an outbreak.
The COVID-19 pandemic has shown that NPIs can be highly effective in controlling the spread of a novel virus when used consistently and at scale [6]. It is also possible that many diseases, other than those already reported, could be controlled by NPIs. Previous studies have indicated that the COVID-19 pandemic and concomitant NPIs led to changes in the transmission dynamics of other notifiable diseases [7–10]. In addition to NPIs, factors such as the effects of vaccination, population immunity, viral variation, and climate change have been suggested to have contributed to these changes [9,11]. While most diseases have shown a clear decline following the COVID-19 pandemic, some diseases have exhibited increases or variable patterns of incidence. Therefore, to better assess the impact of NPIs, conducting long-term follow-up studies on the impact of NPIs on other infectious diseases would be beneficial. Such studies can aid in assessing the sustainability and effectiveness of NPIs in controlling the spread of infectious diseases and can provide valuable insights into the development of future public health strategies, which is important as infectious diseases can have a significant impact on global health, the economy, and social well-being. To understand the epidemiology of other infectious diseases during the pandemic, we aimed to assess the impact of the NPIs implemented during COVID-19 on other nationally notifiable infectious diseases (NNIDs) under surveillance in South Korea.
METHODS
Study design and data source
In South Korea, highly contagious diseases are designated as NNIDs, and the number of patients infected is provided on the Infectious Disease Homepage (https://dportal.kdca.go.kr/pot/index.do), which is operated by the Korea Disease Control and Prevention Agency (KDCA). Among them, the top seven diseases with an average incidence of more than 5,000 cases per year during the study period were selected, namely varicella, carbapenem-resistant Enterobacterales (CRE), mumps, hepatitis C, hepatitis A, scarlet fever, and scrub typhus (Table 1). Tuberculosis and acquired immune deficiency syndrome (AIDS) are also classified as notifiable infectious diseases but are managed under separate systems through specific legislation. Due to the unavailability of daily patient statistics, these two diseases were excluded from the analysis in our research. Long-term data were used to increase the reliability of the trend change analysis, and the number of daily and monthly patients for each disease was obtained for the four years from 2018 to 2021. During this period, annual trend changes for each disease were analyzed, and the point at which the trend in the number of patients changed was identified using the change point detection technique through monthly time-series data. South Korea’s outbreak alert system consists of four levels based on the risk of importation or local transmission: Blue, Yellow, Orange, and Red. The first confirmed case of COVID-19 in South Korea was reported on 20 January 2020. After a cluster infection occurred in Daegu City, the Korean government raised the national crisis alert level to Red on 23 February 2020, meaning that strict NPIs were implemented nationwide. Based on this time point, we investigated whether the change point for each disease was related to the implementation of the NPIs. All procedures were conducted in accordance with the 1975 Declaration of Helsinki, and the study was approved by the Institutional Review Board of Chung-Ang University in Seoul (1041078-20230215-HR-034).
Analysis
We obtained the daily number of patients for each disease and calculated the one-week moving averages of the daily number of patients to calibrate the factor by day of the week. Using these data, the annual difference and the change in trend after COVID-19 for each disease were identified. Next, we calculated monthly data for each disease to analyze trend changes over the entire study period. We employed a change point detection technique to identify the change points for each disease. The analysis consisted of the following steps: Firstly, we conducted the ordinary least squares (OLS)-based cumulative sum (CUSUM) test to determine the presence of change points for each disease. A p value is derived to indicate the significance of the detected change point. Secondly, to determine the optimal number of change points, we used the Bayesian Information Criterion (BIC), with the number of change points associated with the lowest BIC value considered the optimal choice. Finally, we assessed the temporal overlap between the selected change points and the implementation of the NPI, which was accomplished by examining the confidence intervals (CIs) associated with the change points. A CI that spanned the start date of the strictly implemented NPIs (23 February 2020) indicated a significant association between the interventions and the incidence of the disease. All statistical analyses were performed using R (version 3.6.3; R Foundation for Statistical Computing, Vienna, Austria) [12].
RESULTS
The one-week moving averages of the daily number of patients are shown in Figure 1. Varicella exhibited two annual peaks, showing similar patterns in 2018 and 2019, followed by a marked decline after February 2020. These trends persisted until 2021. Scarlet fever maintained a similar trend from mid-2018 until it decreased after February 2020. Mumps cases typically increased around June and mirrored the trends of varicella in 2018 and 2019, but also experienced a steep drop after February 2020, with the overall trend continuing to decline despite a temporary increase in cases in the fall of 2021. In contrast, CRE cases showed a slight upward trend over time, while the number of patients with hepatitis A showed a significant increase in 2019, but no significant changes during the rest of the study period. Hepatitis C and scrub typhus cases did not show significant differences during the study period. Similar trends were noted when the overall incidence of each disease was segmented into pre-NPI and post-NPI periods and analyzed for change rates (Table 2).
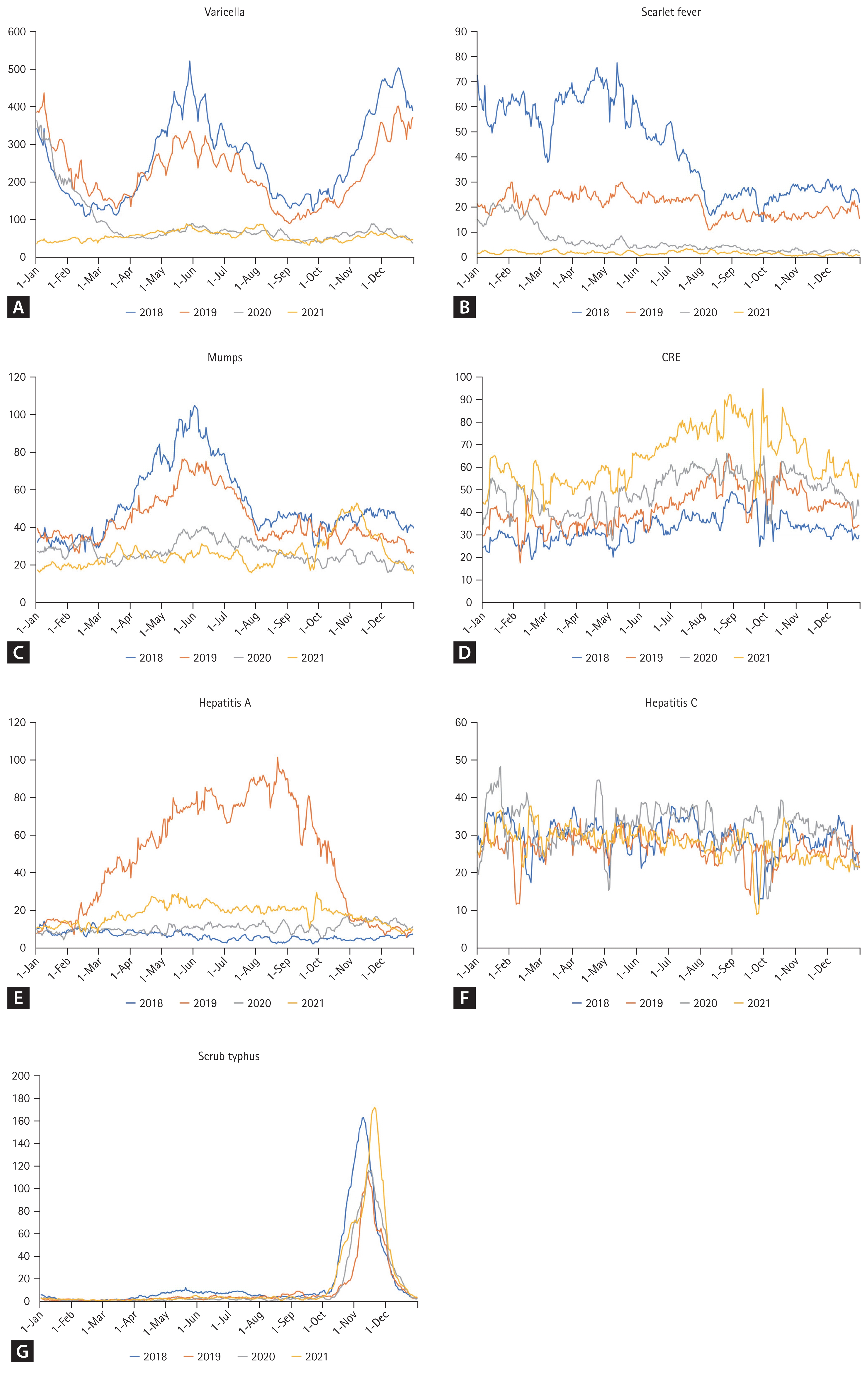
The one-week moving average of the daily number of patients with NNIDs. Varicella, scarlet fever, and mumps exhibit a similar trend. The incidence of these diseases decreased from February 2020 and continued to maintain that trend (A–C). By contrast, CRE showed an increasing trend unrelated to COVID-19 or the implemented NPIs (D). Hepatitis A, hepatitis C, and scrub typhus did not show significant variations throughout the study period, except for a sharp increase in hepatitis A in 2019 (E–G). NNIDs, nationally notifiable infectious diseases; CRE, carbapenem-resistant Enterobacterales; NPI, non-pharmaceutical interventions.
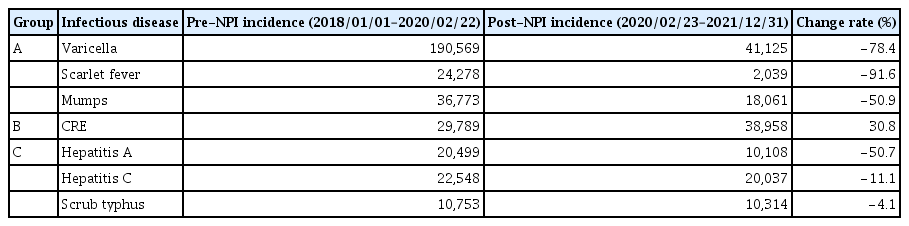
Nationally notifiable infectious diseases and their trends during the COVID-19 pandemic in South Korea
In the analysis using the change point detection technique throughout the entire study period, each disease was categorized into three groups.
Group A: presence of change points coinciding with NPI implementation
Varicella, scarlet fever, and mumps were categorized in Group A. For varicella, a significant change point was detected in the OLS-based CUSUM test (p < 0.001). The optimal number of breakpoints determined using BIC was one, with the corresponding break date identified as January 2020. The 95% CI for the break date ranged from December 2019 to May 2020. Notably, the implementation of the NPIs began on 23 February 2020, suggesting a strong association between NPI implementation and the incidence of varicella (Fig. 2A–C). Similarly, scarlet fever showed significant change points (p < 0.001), with three optimal break dates identified as July 2018, July 2019, and February 2020. Among these, the 95% CI for the break date in February 2020 ranged from January 2020 to March 2020, again coinciding with the period that the NPIs were implemented. For mumps, a single optimal change point was found in July 2019 (p < 0.001) and the corresponding range of the change point also overlapped with NPI implementation (95% CI, June 2019 to February 2020) (Table 3).
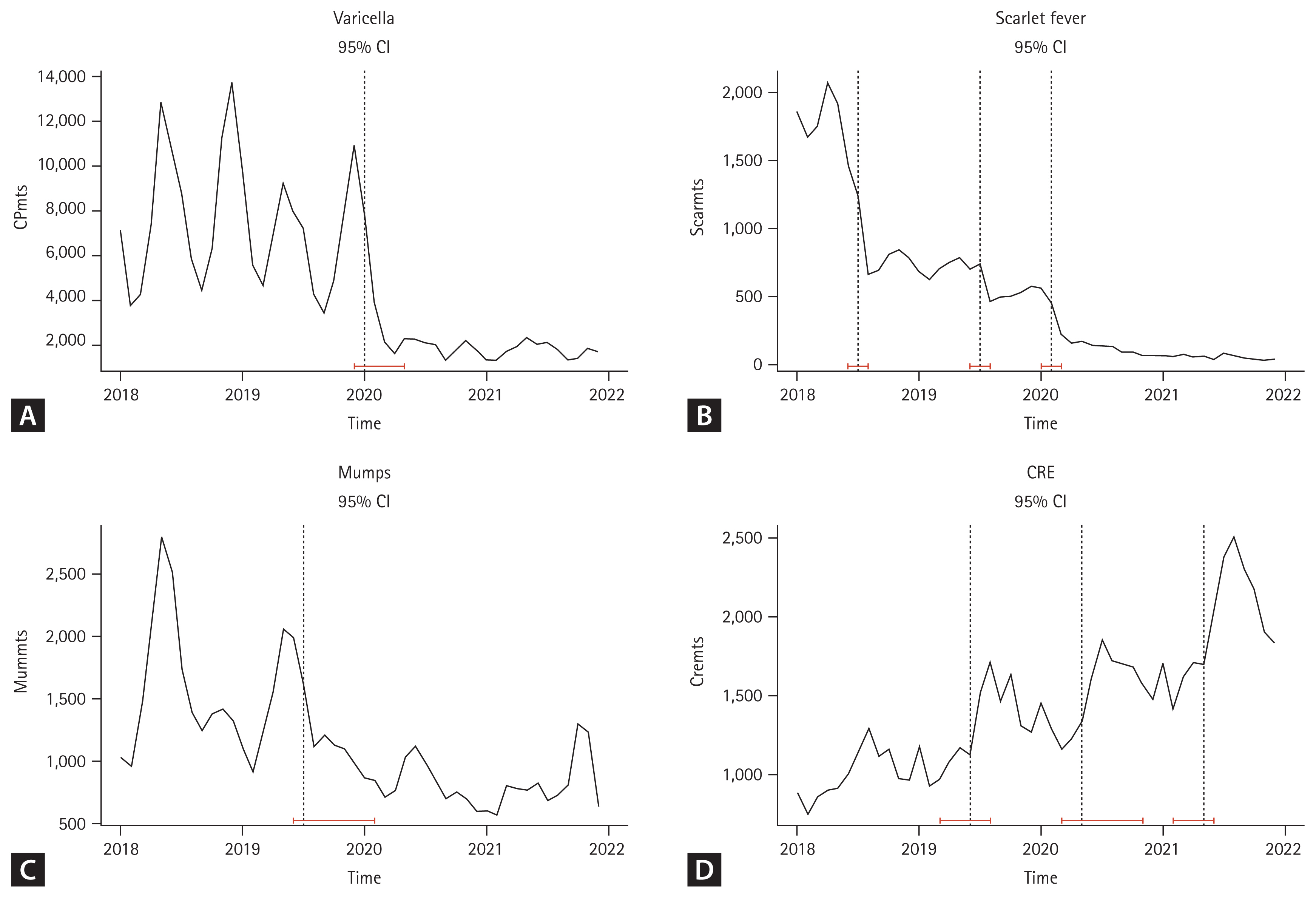
The 95% CI for each change point of the NNIDs (red). The change point detection technique was applied to each disease within the NNID dataset. Based on the result of the OLS-based CUSUM test and BIC, 95% CI was evaluated to determine whether the observed changes coincided with the initiation of NPI. Varicella, scarlet fever, and mumps showed similar results, indicating that the change points were consistent with the NPI implementations (A–C). CRE was in Group B and showed a change point that was not aligned with the implementation of NPI (D). Hepatitis A, hepatitis C, and scrub typhus demonstrated similar results, which did not have significant change points (C). CI, confidence interval; NNIDs, nationally notifiable infectious diseases; OLS-based CUSUM test, ordinary least squares-based cumulative sum test; BIC, Bayesian Information Criterion; NPI, non-pharmaceutical interventions; CRE, carbapenem-resistant Enterobacterales.
Group B: presence of change points not coinciding with NPI implementation
CRE exhibited a significant change point in the OLS-based CUSUM test (p < 0.001). The optimal number of breakpoints with the lowest BIC value was three, and the corresponding break dates were identified as June 2019, May 2020, and May 2021. The 95% CI for the break date closest to the implementation of the NPI ranged from March 2020 to November 2020. However, CRE cases were not associated with NPI implementation, with an increasing trend being observed instead (Fig. 2D, Table 3).
Group C: absence of change points
In Group C, there were no significant change points in the OLS-based CUSUM test for hepatitis A, hepatitis C, or scrub typhus (p = 0.120, 0.399, and 0.733, respectively) with no significant trend changes observed during the entire study period. Therefore, the subsequent steps of BIC and 95% CI were not performed. The detailed results are summarized in Table 3.
DISCUSSION
Our study showed that the trends in the incidence of the NNIDs under surveillance during the COVID-19 pandemic varied depending on the disease. Varicella, scarlet fever, and mumps show a clear decrease in incidence after NPIs were implemented, which is consistent with the results of previously reported studies on these diseases [13,14]. Several factors are commonly considered regarding the occurrence of these diseases. The primary factor is that these diseases are all transmitted via respiratory tract infections. The NPIs implemented during the COVID-19 pandemic could therefore reduce the incidence of other respiratory diseases, thereby reducing overall infection rates [15]. The mechanisms by which NPI might control respiratory tract infections are multifaceted. One major mechanism is reducing the amount of infectious respiratory droplets released into the air, which can be achieved by wearing masks or face coverings that trap respiratory droplets. Another factor involves reducing the duration of close contact between individuals, achievable through social distancing measures. These measures aim to mitigate the transmission of respiratory droplets and prevent the spread of infectious diseases. Hand hygiene is also crucial factor; regular handwashing or the use of hand sanitizer plays a vital role in removing infectious droplets from hands, reducing the risk of transmission. Respiratory etiquette, such as covering the mouth when coughing and sneezing, is also important in preventing the spread of respiratory infections. Lastly, NPIs contribute to reducing the overall burden of respiratory tract infections in the community by reducing infection, which can prevent health systems from becoming overwhelmed, thus maintaining the quality of care and reducing mortality rates.
Another commonly considered factor is that these diseases primarily occur in children. Sun et al. investigated the impact of NPIs on the incidences of vaccine-preventable diseases during the COVID-19 pandemic in eastern China. Their findings indicated that the degree of reduction in disease incidence was age-related, with children experiencing a more significant reduction compared to adults [16]. NPIs may be more effective in controlling disease in children since they are more susceptible to infection than adults, mainly due to immature immune systems, close contact with other children in school and childcare settings, and a tendency to put their hands or objects in their mouths. In addition, parental factors can influence the effectiveness of NPIs by affecting compliance with these interventions. Parents may be more likely to understand the importance of NPIs and to be more motivated to ensure that their children comply with these interventions.
Typical seasonal patterns in varicella and mumps incidence [17] have been disrupted following the COVID-19 pandemic, possibly due to the strict implementation of NPIs. Our findings are in line with a previous study showing a disruption in the seasonality of infectious respiratory diseases during the COVID-19 pandemic in South Korea [14]. Similarly, European data suggest that normal seasonal variations have been interrupted since the onset of the COVID-19 pandemic [18], highlighting the impact of NPIs on seasonal disease transmission. A notable exception occurred in 2021, when mumps cases spiked between October and December. This surge might have been associated with the relaxation of school attendance policies, which permitted a return to in-person classes during the second semester of the relevant academic calendar. However, the resurgence of COVID-19 cases led to a reversal of this decision and the reimplementation of NPIs on 18 October, including social distancing measures, coinciding with changes in mumps case numbers.
The timing of the change point for CRE did not coincide with the onset of the COVID-19 pandemic, as it exhibited a slightly increasing trend. Instead, CRE is more commonly associated with healthcare settings such as intensive care units and long-term care facilities, where transmission occurs through direct contact with contaminated surfaces or the hands of healthcare workers. Previous studies have reported an increase in the incidence of CRE during the COVID-19 pandemic, which could be attributed to several factors. These include the diversion of healthcare resources to the pandemic response, resulting in reduced availability of resources for infection prevention and control measures [19]. The increased use of antibiotics in treating patients with COVID-19 has been implicated in the emergence of antibiotic-resistant bacterial strains, including CRE [20]. Moreover, factors such as poor compliance and inadequate resources can limit the implementation and effectiveness of NPIs in controlling CRE. However, despite these factors, the increase in CRE incidence in South Korea may not be attributed to the impact of NPIs. The actual observed incidence of CRE in South Korea has shown a consistent increasing trend since the mandatory reporting initiated in June 2017, and has been associated with significantly longer hospital stays compared to other Organization for Economic Cooperation and Development (OECD) countries [21,22]. Moreover, other studies that have analyzed the incidence of CRE in South Korea through predictive models using pre-COVID incidence data have reported that the observed data are not significantly different from the predicted values, regardless of the implementation of NPIs [23,24]. Therefore, a more careful approach is required to determine the impact of NPIs on the incidence of CRE.
Hepatitis A, hepatitis C, and scrub typhus showed no significant trend changes during the COVID-19 pandemic. Generally, these diseases are transmitted through non-respiratory routes. Hepatitis A is primarily transmitted through contaminated water or food. According to the Epidemiological Investigation of Infectious Diseases in the Republic of Korea, Annual Report 2019, provided by the Infectious Disease Homepage operated by KDCA, there was a sharp increase in cases of hepatitis A in 2019 in South Korea, likely due to the improper distribution of shellfish during that time [25]. This is consistent with previously reported outbreaks of hepatitis A linked to the consumption of contaminated shellfish [26]. Hepatitis C is transmitted primarily by parenteral routes such as blood transfusion, injecting drug use, contaminated medical equipment, and tattoo needles. Sexual or perinatal transmission is also possible but extremely rare [27]. Hepatitis C is not primarily transmitted through respiratory droplets, so NPIs such as mask-wearing and social distancing are not as effective in preventing its spread. Additionally, the majority of hepatitis C infections occur in individuals who engage in high-risk behaviors, such as injecting drugs, which may not be impacted by COVID-19 or NPIs. As a result, it is likely that the incidence of hepatitis C has not been significantly impacted by NPI. The COVID-19 pandemic may not have had a significant impact on the incidence of scrub typhus because it is mainly transmitted through the bite of infected mites rather than person-to-person transmission. Scrub typhus in South Korea exhibits an atypical pattern of occurrence, with the majority of cases concentrated between October and November in rural areas. It is likely that there was no change point in the incidence of scrub typhus associated with COVID-19 because the occupational aspect, related to farming and exposure to mites found in fields and forests, was not significantly affected by the pandemic.
Despite the significant findings and contributions of this study, it is important to acknowledge and address several limitations. First, the analysis relied on retrospective data without a control group, making it difficult to attribute the observed changes solely to NPIs. Second, this study focused primarily on associations and trends, without establishing a causal relationship between NPIs and disease incidence. Moreover, our study only focused on NNIDs in South Korea and may not fully capture the epidemiology of other diseases or regions. The effectiveness of NPIs may also vary depending on population density, cultural practices, healthcare infrastructure, and other contextual factors, which were not fully considered in the analysis. These factors should be considered when generalizing our findings to other settings. Additionally, tuberculosis and AIDS were excluded from our analysis. Tuberculosis is the second most prevalent disease after varicella, with an average of 22,130 cases per year between 2018 and 2021, but we were unable to include it due to insufficient data available for analysis. Finally, several factors other than NPIs that could influence the incidence of these infectious diseases were not assessed in this study. These include changes in immunity, the effects of vaccination, climate change, and altered healthcare-seeking behaviors and surveillance capacity [9–11]. Taken together, these limitations highlight the need for further research to comprehensively understand the impact of NPIs on infectious diseases.
In conclusions, this study highlighted the effectiveness of NPIs in controlling respiratory tract infections, as evidenced by the decline in varicella, mumps, and scarlet fever cases following the NPI implementations during the COVID-19 pandemic in South Korea. Understanding the differential impact of NPI on various infectious diseases is crucial for optimizing public health interventions. These findings contribute to the knowledge base on the sustainability and effectiveness of NPI in controlling the spread of infectious diseases and inform the development of comprehensive public health policies and strategies.
KEY MESSAGE
1. The implementation of NPIs to control COVID-19 was associated with a significant decline in the incidence of certain infectious diseases such as varicella, scarlet fever, and mumps in South Korea.
2. However, the incidence of CRE showed an increasing trend unrelated to NPI implementation, and hepatitis A, hepatitis C, and scrub typhus did not show significant changes during the NPI period.
3. The findings of the study suggest that NPIs effectively controlled infectious diseases, particularly those transmitted through respiratory infections.
Notes
CRedit authorship contributions
Hyun-Jin Lee: conceptualization, data curation, formal analysis, writing - original draft, writing - review & editing, visualization; Beom Seuk Hwang: methodology, investigation, formal analysis, writing - review & editing, visualization; Seong Ho Im: methodology, investigation, formal analysis; Seog-Kyun Mun: conceptualization, methodology, writing - review & editing, visualization, supervision; Munyoung Chang: conceptualization, investigation, data curation, writing - original draft, writing - review & editing, supervision
Conflicts of interest
The authors disclose no conflicts.
Funding
This research was supported by the Chung-Ang University Research Grants in 2021. The authors have no other funding, financial relationships, or conflicts of interest to disclose.
Data availability statement
The data used in this study are available at the Infectious Disease Homepage (https://dportal.kdca.go.kr/pot/index.do) operated by KDCA.