Current evidence for prognostic benefit of intravascular imaging-guided percutaneous coronary intervention in chronic total occlusion intervention
Article information
Abstract
Although percutaneous coronary intervention (PCI) for chronic total occlusion (CTO) has been increasing in recent years, CTO PCI is still one of the most challenging procedures with relatively higher rates of procedural complications and adverse clinical events after PCI. Due to the innate limitations of invasive coronary angiography, intravascular imaging (IVI) has been used as an adjunctive tool to complement PCI, especially in complex coronary artery disease. Considering the complexity of CTO lesions, the role of IVI is particularly important in CTO intervention. IVI has been a useful adjunctive tool in every step of CTO PCI including assisted wire crossing, confirmation of wire location within CTO segment, and stent optimization. The meticulous use of IVI has been one of the greatest contributors to recent progress of CTO PCI. Nevertheless, studies evaluating the role of IVI during CTO PCI are limited. The current review provides a comprehensive overview of the mechanistic advantages of IVI in CTO PCI, summarizes previous studies and trials, and presents future perspective of IVI in CTO PCI.
INTRODUCTION
Despite the increase of procedural volume of chronic total occlusion (CTO) percutaneous coronary intervention (PCI), CTO PCI is still considered one of the most challenging procedures, even with the development of new devices and advanced techniques [1]. In fact, the unique and complex features of CTO lesion, such as severe and diffuse plaque burden, negative remodeling of the vessel, and heavy calcification, are major obstacles for successful CTO PCI [2].
Due to the innate limitations of invasive coronary angiography, intravascular imaging (IVI) devices that can provide continuous cross-sectional image of a vessel, such as intravascular ultrasound (IVUS) and optical coherence tomography (OCT), were introduced to complement the procedure and improve clinical outcomes [3]. The use of IVI in CTO PCI, along with technical advancements, is acknowledged as a key contributor to recent progress and increased success rate of CTO PCI. Despite the potential advantages of IVI in CTO PCI, the prognostic impact of IVI-guidance and optimization in CTO PCI has been unclear due to limited evidence from randomized controlled trials (RCTs). Although several RCTs demonstrated the prognostic benefit of IVI in complex coronary artery lesions other than CTO [4–7], only limited evidence exists regarding the prognostic benefit of IVI in CTO PCI [8–10]. Therefore, the current review consists of three parts: 1) Potential benefit of IVI in CTO Interventions, 2) Current evidences, and 3) Future perspectives and ongoing trials.
POTENTIAL BENEFIT OF IVI IN CTO INTERVENTIONS
The fundamental purpose of IVI in PCI is for procedural optimization [3]. For this purpose, IVI can be used in both pre- and post-PCI phases [11]. In pre-PCI phase, IVI is used to assess lesion characteristics and to determine strategy of lesion preparation, stent size, and landing zone accordingly. In post-PCI phase, IVI is used to check whether stent is optimally implanted and for the presence of acute complications such as edge dissection or plaque/thrombus protrusion in the stented segment. Considering the complexity of CTO lesions, the use of IVI for these general purposes is even more emphasized. In addition, IVI can be used for identifying entry of CTO lesions, IVUS-guided puncture in case of angiographically ambiguous proximal cap, guidance in retrograde re-entry attempts, and confirmation of wire location (intraplaque vs. extraplaque tracking) after crossing [12]. Since OCT requires injection of contrast agents for image acquisition, which may extend dissection planes, IVUS is the preferred modality in the pre-PCI phase [13]. However, OCT can be useful to assess stent-related problems such as underexpansion, malapposition, or edge dissection and to optimize the stented segment(s).
Guiding wire crossing
Ambiguous proximal cap in CTO lesion is estimated to account for approximately 30% of CTO PCI and is one of the major causes of wire crossing failure [14]. The low success rate in wire crossing through ambiguous proximal cap can be overcome through IVUS guidance. IVUS catheter is advanced to the side branch that diverges at the site of proximal cap of CTO lesion (Fig. 1). IVUS imaging can be obtained simultaneous real-time or intermittently to guide the location of proximal cap and guide wire [12]. Furthermore, IVUS image can provide additional information about plaque characteristics of the proximal cap, which can influence the choice of guide wire.

IVUS-guided puncture in angiographically ambiguous proximal cap of CTO. A 69-year-old patient with ischemic cardiomyopathy showed depressed left ventricular systolic function (ejection fraction 24% on echocardiography) and CTO in left anterior descending artery (LAD). (A) Proximal stump of CTO had ambiguous cap in angiography. (B) IVUS pullback from diagonal branch showed totally occluded LAD (white arrowhead). (C) The corresponding point was marked based on IVUS information (white arrowhead). (D) Gaia Next 2nd wire (Asahi Medical, Japan) was used to puncture the proximal cap which was identified by IVUS. Gaia Next 2nd wire crossed into 2nd diagonal branch. Under support of dual lumen microcatheter (Sasuke™; Asahi Medical, Japan), Miracle 6 wire successfully crossed into the distal cap of CTO segment. (E) Xience Skypoint (Abbott Vascular USA) 2.75 × 38 mm at mid to distal LAD and Xience Skypoint 3.5 × 48 mm at proximal to mid LAD were implanted and optimized using IVUS. CTO, chronic total occlusion; IVUS, intravascular ultrasound; LAD, left anterior descending artery.
IVUS can also facilitate CTO lesion crossing in re-entry techniques (Fig. 2). With the emergence of advanced wire crossing techniques such as subintimal tracking and re-entry (STAR) and reverse controlled antegrade and retrograde tracking (CART), IVUS can play a key role in these techniques [13]. In these crossing techniques, IVUS can confirm the position of the guide wire (intraplaque vs. extraplaque) and provide information about vessel size to determine the appropriate balloon size in reverse CART to increase successful wire crossing and reduce the risk of perforation [15].
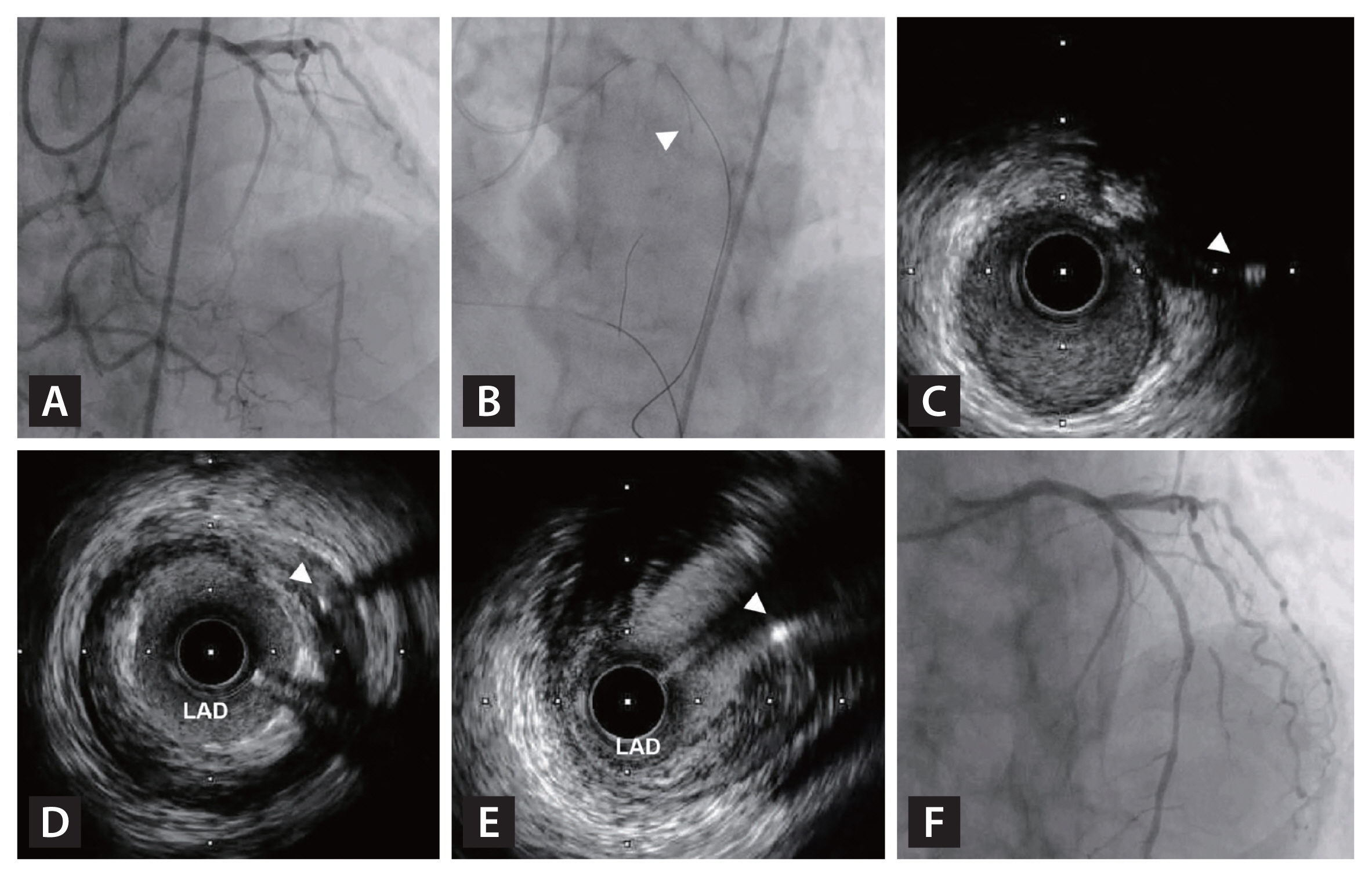
IVUS-guidance in retrograde re-entry into CTO. (A) A 74-year-old patient presented with CCS class III stable angina and showed CTO in LAD. (B) Based on angiographically ambiguous proximal cap and entry point, initial retrograde approach was attempted using Suoh 03 wire and Caravel microcatheter (Asahi Medical, Japan). (C) Simultaneous IVUS imaging in the septal branch showed retrograde Ultimate Bros 3 wire (white arrowhead) was located near proximal cap of CTO segment. (D) However, Ultimate Bros 3 (white arrowhead) could not cross into the true lumen of proximal LAD. (E) Under simultaneous IVUS imaging, re-attempt using Conquest Pro 12 (Asahi Medical, Japan) succeeded to cross into the true lumen of proximal LAD (white arrowhead). (F) Xience Skypoint 3.5 × 33 mm (Abbott Vascular, USA) was successfully implanted at proximal LAD. CCS, Canadian Cardiovascular Society; CTO, chronic total occlusion; IVUS, intravascular ultrasound; LAD, left anterior descending artery.
Intraplaque vs. extraplaque tracking
After successful wire crossing, IVI provides information about wire location (Fig. 3). Previous study presented that operator-reported tracking showed discordance with IVUS-detected actual wire tracking [16]. When interrogated with IVUS, unintended extraplaque tracking was noticed in nearly 90% in re-entry techniques and about 30% in antegrade or retrograde wiring in this study [16]. Extraplaque tracking may lead to vessel injury such as intramural hematoma and dissection, and side branch occlusion after recanalization [12,16]. Consequently, accurate identification of wire tracking has the possibility to reduce complications and improve clinical outcomes.
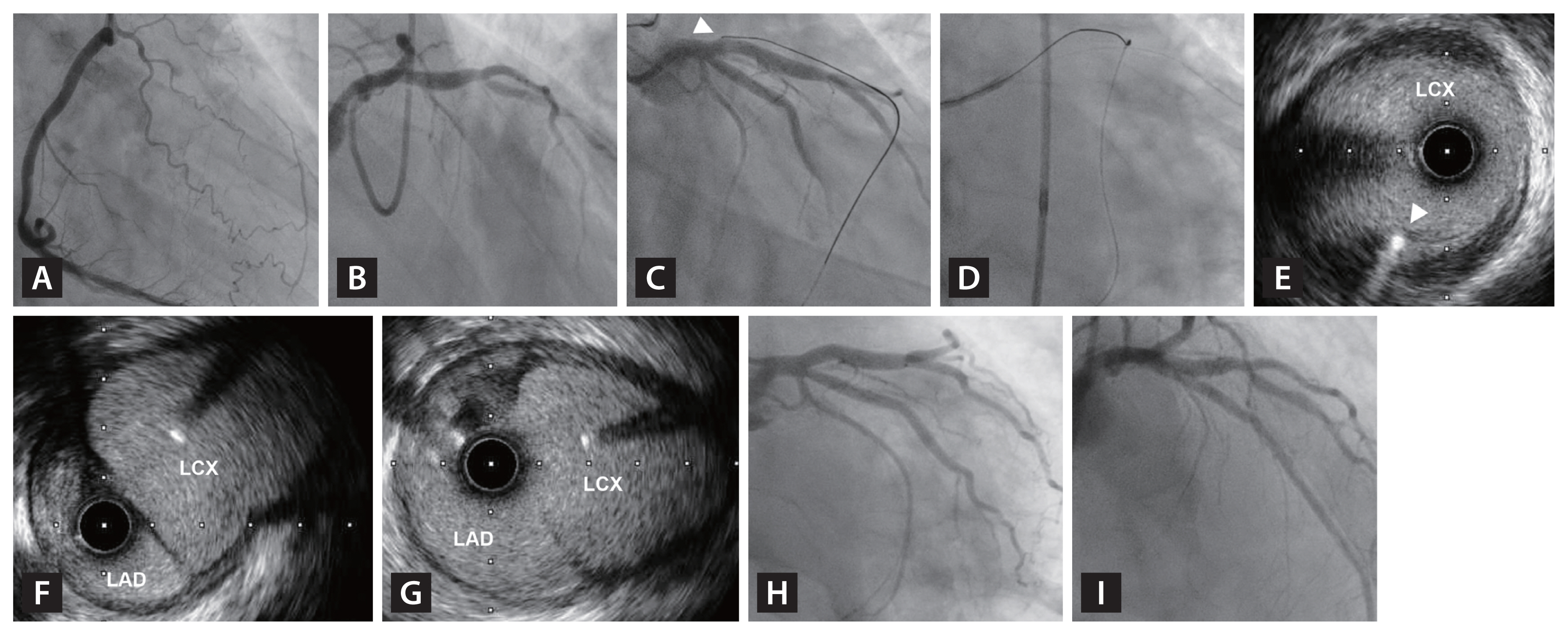
Confirmation of wire location (intraplaque vs. extraplaque tracking) by IVUS after wire crossing in CTO. A 61-year-old patient with ischemic cardiomyopathy showed mildly depressed left ventricular systolic function (ejection fraction 45% on echocardiography) and had a CTO lesion in previously implanted stent at LAD ostium 12 years ago. (A, B) There was no identifiable entry point or proximal cap in angiography of left coronary artery. (C) Initial retrograde crossing was attempted using Suoh 03 wire under Cosair Pro XS microcatheter (Asahi Medical, Japan). Arrowhead indicates proximal stump. (D) crossing of proximal cap was done with Ultimate Bros 3 wire (Asahi Medical, Japan). (E) After reentry into left main, IVUS pullback was performed in LCX, which showed intraplaque position of the wire (white arrowhead). (F) After externalization and exchange of retrograde wire, IVUS showed slightly expanded intraplaque false lumen. Multiple attempts of reentry using different wires failed to engage true lumen, therefore, cutting balloon angioplasty was performed to fenestrate between false and true lumen. (G) After 3.5 × 10 mm cutting balloon (Flaxtome; Boston Scientific, USA) angioplasty, successful fenestration of both lumens was observed in IVUS. (H, I) Synergy XD 3.5 × 20 mm (Boston Scientific, USA) was successfully implanted at left main to proximal LAD. CTO, chronic total occlusion; IVUS, intravascular ultrasound; LAD, left anterior descending artery; LCX, left circumflex artery.
Lesion preparation, stent planning, and post-stent optimization
Principal roles of IVI during PCI of complex coronary artery lesions can also be applied to CTO PCI [11]. Prior to stent deployment, IVI is crucial to assess reference vessel size, lumen area, lesion length, and plaque characteristics, including composition and negative or positive remodeling [17]. This information can influence lesion preparation and plaque modification strategy. In addition, it can be used to determine the optimal stent size, length, and appropriate landing zone (Fig. 4). After stent implantation, IVI can be used to check for stent optimization and for the presence of acute procedure-related complications. Stent optimization is composed of sufficient stent expansion, absence of major stent malapposition to the vessel wall, and absence of major edge dissection (Fig. 5, 6) [7,11]. Successful achievement of stent optimization has been reported to be associated with better clinical outcomes [7,18].
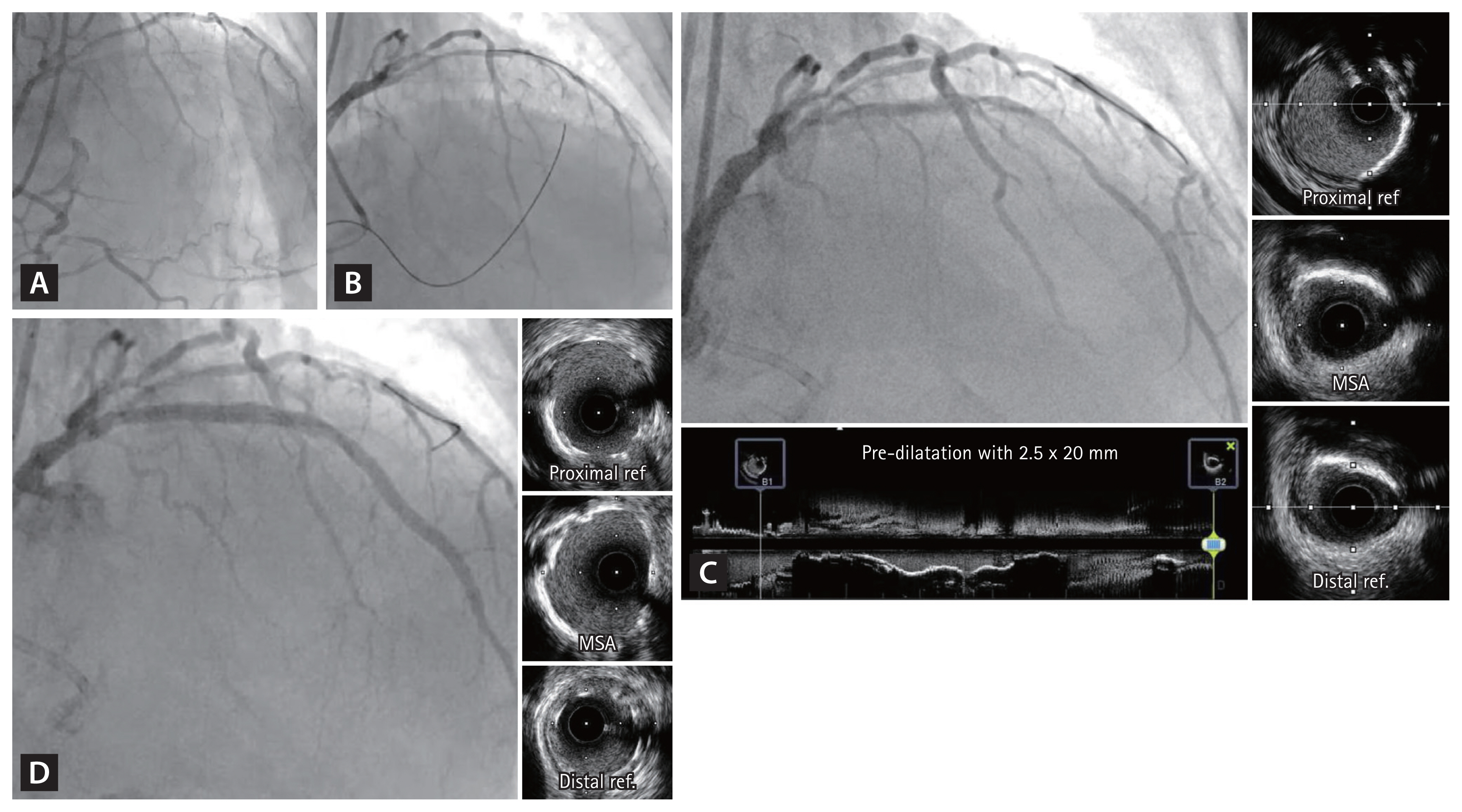
Determination of lesion preparation strategy, stent size, and landing zone by IVUS. A 57-year-old patient presented with CCS class II stable angina and positive exercise stress echocardiography and preserved ejection fraction. (A) Proximal LAD CTO with good collateral flow from RCA was observed. Heavily calcified proximal LAD segment was noted, and Conquest Pro 12 wire could not penetrate the proximal cap. Therefore, retrograde approach using Fielder XT-R (Asahi Medical, Japan) and Caravel microcatheter, which successfully crossed into distal cap of LAD CTO segment. (C) CTO segment was crossed by Gaia Next 2nd wire and IVUS pullback after pre-dilated by semi-compliant 2.5 × 20 mm balloon showed heavy calcification throughout proximal to mid LAD. Based on IVUS findings, 2.75 × 20 mm non-compliant balloon additionally dilated the CTO segment. (D) After implantation of Xience Skypoint 3.5 × 38 mm and 3.25 × 32 mm stents, additional stent optimization was performed using 3.5 × 20 mm non-compliant balloon. Final IVUS showed well expanded stents with good stent apposition without edge dissection or plaque protrusion. CCS, Canadian Cardiovascular Society; CTO, chronic total occlusion; IVUS, intravascular ultrasound; LAD, left anterior descending artery; MLA, minimum lumen area; MSA, minimum stent area; RCA, right coronary artery.

Stent optimization by OCT. A 53-year-old patient presented with new onset exertional chest pain. (A) Coronary angiography showed short-segment CTO at proximal LAD with good collateral flow from RCA. (B) Initial anterograde cross was attempted with Gaia Next 2nd wire, and retrograde angiography confirmed the successful true lumen crossing of the wire. (C) After pre-dilatation using 3.0 × 15 mm scoring balloon, OCT showed large amount of lipid plaque in the CTO segment. (D, E) Synergy Megatron 3.5 × 28 mm (Boston Scientific, USA) was implanted at proximal LAD and proximal edge of the stent was further dilated using 4.0 × 15 mm non-compliant balloon. (F) Final OCT showed good expansion and apposition of the stent. CTO, chronic total occlusion; LAD, left anterior descending artery; OCT, optical coherence tomography; RCA, right coronary artery.

Evaluation of acute complications after stent implantation. (A) A 65-year-old patient with stable angina showed severe stenosis at proximal LAD with heavy calcification. After rotablation, Synergy XD 3.5 × 28 mm was implanted. (B) Post-stenting coronary angiography showed contrast leakage at proximal edge of the stent (white arrowhead). (C) IVUS imaging showed huge medial dissection (white arrowhead). The dissection was covered with additional stenting. (D, E) A 52-year-old patient with unstable angina had critical stenosis at mid LAD. Xience Sierra 3.5 × 24 mm was implanted at mid LAD. (F) Post-stenting IVUS imaging showed minor intimal dissection (white arrowhead). Because the dissection was confined to the intima and its length was short, medical treatment was chosen. IVUS, intravascular ultrasound; LAD, left anterior descending artery.
CURRENT EVIDENCE
Several non-randomized studies and RCTs have evaluated the effectiveness of IVI compared to angiography alone in CTO PCI. In this section, results of previous studies are summarized. Although there have been limited number of RCTs, study-level meta-analysis revealed the prognostic benefit of IVI-guided PCI than angiography-guided PCI in CTO procedure.
Non-randomized study for prognostic benefit of IVI-guided CTO PCI
Non-randomized studies that evaluated the role of IVI in CTO PCI are summarized in Table 1 [16,19–26]. Most studies were retrospective analyses. In terms of IVI modality, 6 studies used IVUS, 1 study used OCT, and the 2 remaining studies used both IVUS and OCT. In these retrospective studies, clinical outcomes assessments were limited to index hospitalization and showed no significant differences in in-hospital mortality between the IVI-guided and angiography- guided PCI groups. Maknojia et al. analyzed 33,345 patients from Nationwide Inpatient Sample (NIS) database who underwent CTO PCI from 2015 to 2018 [25]. In this study, only 8.8% of the total population used IVI for CTO PCI, and in-hospital mortality was similar between patients who did and did not receive IVI during CTO PCI (1.5% vs. 1.3%, p = 0.195). Another study from the PROGERSS-CTO registry which enrolled 8,771 patients from 39 centers in a retrospective manner, the use of IVUS was associated with higher technical (99% vs. 96%, p < 0.001) and procedural success rates (96% vs. 95%, p = 0.002). Unfortunately, these results did not translate into better prognosis, and in-hospital major adverse cardiovascular events (MACE) were comparable (2.04% vs. 1.62%, p = 0.176) between the IVI-guided PCI group and angiography-guided PCI group [26]. Similarly, Kalogeropoulos et al. [24] compared a composite endpoint of all-cause death, myocardial infarction, and target-vessel revascularization during median follow-up of 49 months among propensity matched 364 patients, but there was no significant difference in the risk of the composite endpoint between IVUS-guided and angiography-guided PCI group (13.7% vs. 15.9%, log-rank p = 0.67). However, as these studies had non-randomized design with short follow-up duration, the results should be interpreted with caution. Indeed, patients with more complex CTO lesions were more likely to use IVI in these studies. For example, patients in the IVI-guided PCI group had higher J-CTO score than angiography-guided PCI group, which limits the interpretation of these results [21,26].
Randomized trials for prognostic benefit of IVI-guided CTO PCI
Of the 3 RCTs that compared the effectiveness of IVI in CTO PCI to date, 2 RCTs showed that IVI-guided PCI improved clinical outcomes than angiography-guided PCI, and the other trial showed significantly better angiographic results with comparable clinical outcomes between the 2 groups (Table 2) [8–10].
In the AIR-CTO (Angiographic and clinical comparisons of intravascular ultrasound-versus angiography-guided drug-eluting stent implantation for patients with chronic total occlusion lesions) trial, 230 patients were randomly assigned to either IVUS-guided or angiography-guided PCI groups [8]. IVUS-guided PCI significantly reduced in-stent late lumen loss compared to angiography-guided PCI (0.28 ± 0.48 mm vs. 0.46 ± 0.68 mm, p = 0.025), however, the rates of MACE (a composite of all-cause death, myocardial infarction, repeat revascularization, or stent thrombosis) at 2 years were only numerically lower in the IVUS-guided PCI group than the angiography-guided PCI group without statistical significance (21.7% vs. 25.2%, p = 0.641). However, it should be noted that this trial was not powered to show differences in clinical endpoint. In the CTO-IVUS (Chronic Total Occlusion InterVention with drug-eluting Stents guided by IVUS) trial, 402 patients were randomly assigned to IVUS-guided PCI or angiography-guided PCI groups. At 1 year, the risk of MACE (a composite of cardiac death, myocardial infarction, and target-vessel revascularization) was significantly lower in the IVUS-guided PCI than the angiography-guided PCI (2.6% vs. 7.1%; hazard ratio [HR] 0.35; 95% confidence interval [CI] 0.13–0.97; p = 0.035) [9].
The recently published RENOVATE-COMPLEX-PCI (The Randomized Controlled Trial of Intravascular Imaging Guidance versus Angiography-Guidance on Clinical Outcomes after Complex Percutaneous Coronary Intervention) demonstrated for the first time that IVI-guided PCI for complex coronary artery lesions is superior to angiography-guided PCI in reducing the risk of target vessel failure (a composite of cardiac death, target-vessel myocardial infarction and target-vessel revascularization) among 1,639 patients with complex coronary artery lesions [7].
A prespecified substudy of the RENOVATE-COMPLEX-PCI exclusively evaluated 319 patients who underwent CTO PCI [10]. Among patients with CTO, angiographic and procedural characteristics including J-score (median J-CTO score: 2.0 [1.0–3.0] vs. 2.0 [1.0–3.0], respectively, for IVI-guided and angiography-guided PCI; p = 0.755) were similar between the IVI and angiography-guided PCI groups. At a median follow-up of 2.1 years (interquartile range 1.1–3.0 yr), the risk of target vessel failure was not significantly different between patients with CTO and non-CTO lesions (7.6% vs. 9.6%; HR 0.797; 95% CI 0.505–1.258, p = 0.331). Among patients with CTO, the risk of target vessel failure was significantly lower in the IVI-guided PCI group than in the angiography-guided PCI group (5.0% vs. 13.5%; HR 0.303; 95% CI 0.130–0.710, p = 0.006). When patients were classified according to the presence of CTO and the use of IVI, the risk of TVF was significantly different among the 4 groups, and the risk of target vessel failure was the highest in CTO patients treated by angiography-guided PCI and the lowest in CTO patients treated by IVI-guided PCI (Fig. 7). These results suggest that among various complex coronary artery lesions subsets, the efficacy of IVI-guided PCI is relatively higher in CTO intervention.
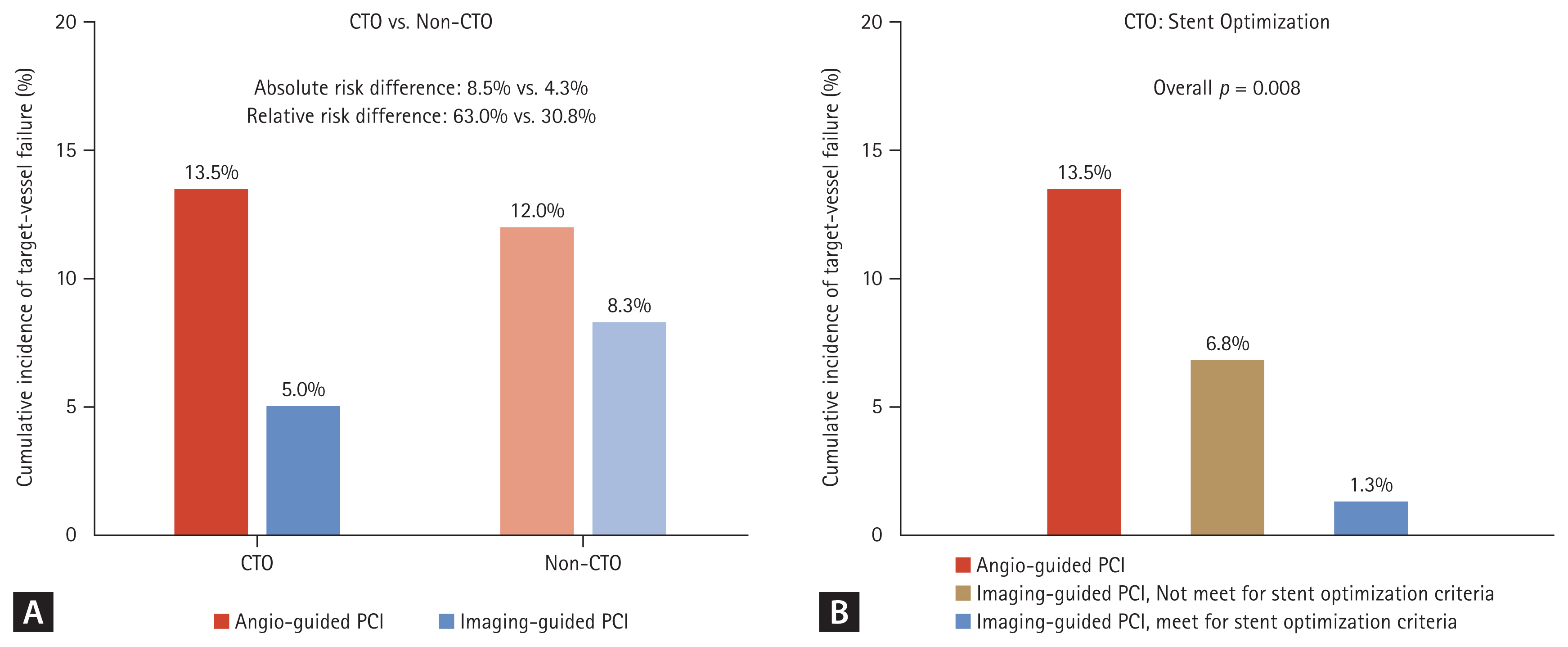
Prognostic impact of intravascular imaging-guided chronic total occlusion percutaneous coronary intervention in RENOVATE-COMPLEX-PCI trial. (A) The risk of target-vessel failure was compared according to the use of intravascular imaging and lesion type (CTO vs. non-CTO complex coronary artery lesions). (B) In patients with CTO lesions, the intravascular imaging-guided PCI group was sub-divided according to stent optimization, and the risk of target-vessel failure was compared with the angiography-guided PCI group. CTO, chronic total occlusion; PCI, percutaneous coronary intervention.
Furthermore, the risk of target vessel failure was shown to gradually increase from patients with IVI–guided PCI and stent optimization, patients with IVI–guided PCI but no stent optimization, and patients with angiography-guided PCI (1.3% vs. 6.8%, 13.5%, overall p = 0.008) (Fig. 7). These results imply that both IVI use and achieving stent optimization in CTO PCI is important for patient’s prognosis. In addition, the benefit of IVI was consistently found in subgroups divided by lesion complexity assessed by J-CTO score (Fig. 8).
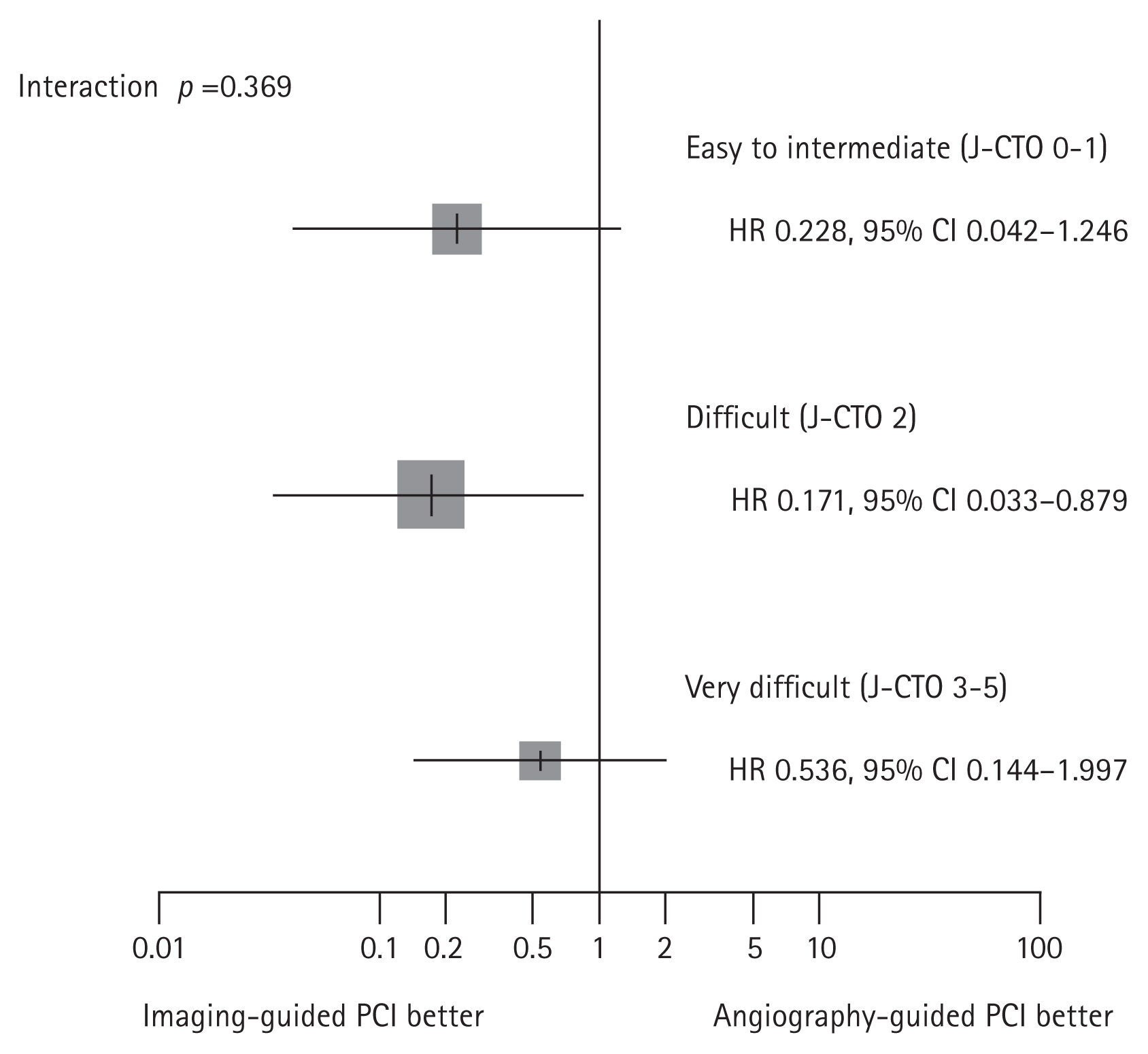
The risk of target-vessel failure according to lesion complexity by J-CTO score in the RENOVATE-COMPLEX-PCI trial. The calculated J-CTO score reflects the severity of CTO lesion: 1) blunt stump at entry; 2) calcification within the CTO segment; 3) bending > 45°; 4) occlusion length ≥ 20 mm; 5) re-attempted CTO procedure. J-CTO 0 to 1 presents easy to intermediate lesion. J-CTO 2 is the more difficult lesion and J-CTO score 3 or higher is considered as the most difficult complex lesion. Regardless of the severity, reduced risk of target-vessel failures was consistently shown in the imaging-guided PCI group than angiography-guided PCI group. CI, confidence interval; CTO, chronic total occlusion; HR, hazard ratio; J-CTO, Japanese-CTO; PCI, percutaneous coronary intervention.
Meta-analysis of CTO PCI using RCTs
Since previous RCTs showed conflicting results regarding prognostic impact of IVI-guided PCI in CTO intervention [8–10], study-level meta-analysis was performed by the current authors. A total of 951 patients who were randomly assigned to IVI-guided PCI (n = 536) or angiography-guided PCI (n = 415) were evaluated. In random effects model, IVI-guided PCI had significantly lower risk of a composite outcome of cardiac death, myocardial infarction, and target vessel revascularization than angiography-guided PCI in CTO intervention (relative risk 0.49; 95% CI 0.25–0.95; p = 0.034). Individual components of the composite outcomes showed similar trends, with statistical significance only met in target vessel revascularization (Fig. 9).

Meta-analysis of intravascular imaging-guided PCI in patients with CTO. Forest plots comparing (A) composite outcome of cardiac death, myocardial infarction, and target-vessel revascularization, (B) cardiac death, (C) myocardial infarction, and (D) target-vessel revascularization after intravascular imaging or angiography-guided PCI groups. Relative risks for individual trials are represented by squares, and 95% CI are represented by horizontal lines. Pooled estimates and their 95% CI are represented by red diamonds. CI, confidence interval; CTO, chronic total occlusion; PCI, percutaneous coronary intervention; RR, relative risk.
FUTURE PERSPECTIVES AND ONGOING TRIALS
Currently, there are 2 ongoing studies with a relatively large number of patients regarding the impact of IVI in CTO PCI (Table 3). CRUISE-CTO trial (To Evaluate Whether IVUS-guided Drug-eluting Stent Implantation Leads to Better Clinical Outcomes Compared to Conventional Angiography in the Treatment of Chronic Complete Occlusion Disease, NCT04944615) will enroll 1,448 patients with CTO and compare the risk of MACE (a composite of death, myocardial infarction, definite or probable stent thrombosis, and clinically-driven target vessel revascularization) between IVUS-guided PCI vs. angiography-guided PCI. FWCTO registry (CTO Registry From Insight of IVUS in Fuwai Hospital, NCT03461367) is a prospective observational study and will enroll 5,000 patients who underwent CTO PCI with or without IVUS-guidance and optimization.
In addition, there are multiple RCTs which will evaluate the prognostic impact of IVUS or OCT-guided PCI compared with angiography-guided PCI in all-comers with complex coronary artery lesions including CTO. The IMPROVE (IMPact on Revascularization Outcomes of IVUS Guided Treatment of Complex Lesions and Economic Impact, NCT04221815) and IVUS-CHIP (Intravascular Ultrasound Guidance for Complex High-risk Indicated Procedures, NCT04854070) trials will enroll all-comers with complex coronary artery lesions and evaluate IVUS-guided PCI. The OCCUPI (Optical CoherenCe Tomography-gUided Coronary Intervention in Patients With Complex lesIons: a Randomized Controlled Trial, NCT03625908) is currently ongoing and will compare OCT-guided PCI versus angiography-guided PCI in similar populations including CTO.
These trials are expected to provide more definite answers regarding the prognostic benefit of IVI in CTO PCI. Moreover, it would be a very interesting and clinically relevant topic to evaluate whether CTO PCI, when optimally performed using IVI, can improve patient prognosis compared to medical treatment alone. Simultaneously, efforts should be made to educate and train cardiac catheterization laboratory staff and physicians in the use of IVI in CTO PCI to promote broader use and improve outcomes for their patients.
CONCLUSION
IVI, especially IVUS, can be used to facilitate guide wire crossing and stent optimization in CTO PCI. Meta-analysis of RCTs conducted to date showed that IVI-guided PCI in CTO lesions significantly improves patients’ prognosis than angiography- guided PCI, supporting the use of IVI in CTO PCI. Further large-scaled trials are warranted to consolidate the benefit of IVI-guidance and procedural optimization in CTO PCI and to facilitate the widespread use of IVI in CTO PCI.
Notes
CRedit authorship contributions
David Hong: conceptualization, methodology, resources, investigation, formal analysis, validation, software, visualization; Sung Eun Kim: conceptualization, methodology, resources, investigation, formal analysis, validation, software, writing - original draft, writing - review & editing, visualization, supervision, project administration; Seung Hun Lee: conceptualization, methodology, formal analysis, validation, writing - original draft, writing - review & editing, visualization, supervision; Seung-Jae Lee: conceptualization, resources, investigation, software; Jong-Young Lee: conceptualization, formal analysis, visualization, supervision; Sang Min Kim: conceptualization, formal analysis, supervision; Sang Yeub Lee: writing - original draft, supervision; Woochan Kwon: conceptualization, methodology, resources, formal analysis, validation, software, visualization, supervision; Ki Hong Choi: conceptualization, supervision; Taek Kyu Park: validation, supervision; Jeong Hoon Yang: conceptualization, supervision; Young Bin Song: conceptualization, methodology, resources, investigation, data curation, formal analysis, validation, software, writing - original draft, writing - review & editing, visualization, supervision, project administration, funding acquisition; Seung-Hyuk Choi: conceptualization, formal analysis, visualization, supervision, funding acquisition; Hyeon-Cheol Gwon: conceptualization, formal analysis, visualization, supervision; Joo-Yong Hahn: conceptualization, methodology, resources, investigation, formal analysis, validation, software, writing - original draft, writing - review & editing, visualization, supervision, project administration, funding acquisition; Joo Myung Lee: conceptualization, methodology, resources, investigation, data curation, formal analysis, validation, software, writing - original draft, writing - review & editing, visualization, supervision, project administration, funding acquisition
Conflicts of interest
Dr. Joo Myung Lee received an Institutional Research Grant from Abbott Vascular, Boston Scientific, Philips Volcano, Terumo Corporation, Zoll Medical, Donga-ST, and Yuhan Pharmaceutical. Prof. Joo-Yong Hahn received an Institutional Research Grant from National Evidence- based Healthcare Collaborating Agency, Ministry of Health & Welfare, Korea, Abbott Vascular, Biosensors, Boston Scientific, Daiichi Sankyo, Donga-ST, Hanmi Pharmaceutical, and Medtronic Inc. Prof. Hyeon-Cheol Gwon received an Institutional Research Grant from Boston Scientific, Genoss, and Medtronic Inc. All other authors declare that there are no competing interests to declare.
Funding
The RENOVATE-COMPLEX-PCI trial is investigator-initiated with grant support from Abbott Vascular and Boston Scientific. Other than providing financial support, the sponsors were not involved with protocol development or the study process, including site selection, study management, data collection, and analysis of the results.