How lessons learned from the 2015 Middle East respiratory syndrome outbreak affected the response to coronavirus disease 2019 in the Republic of Korea
Article information
Abstract
The Republic of Korea (ROK) experienced a public health crisis due to Middle East respiratory syndrome (MERS) in 2015 and is currently going through the coronavirus disease 2019 (COVID-19) pandemic. Lessons learned from the disastrous MERS outbreak were reflected in the preparedness system, and the readiness capabilities that were subsequently developed enabled the country to successfully flatten the epidemic curve of COVID-19 in late February and March 2020. In this review, we summarize and compare the epidemiology and response of the ROK to the 2015 MERS outbreak and the COVID-19 epidemic in early 2020. We emphasize that, because further COVID-19 waves seem inevitable, it is urgent to develop comprehensive preparedness and response plans for the worst-case scenarios of the COVID-19 pandemic. Simultaneously strengthening healthcare capacity to endure the peak demand and implementing smart strategies to sustain social distancing and public hygiene are necessary until safe and effective therapeutics and vaccines against COVID-19 are available.
INTRODUCTION
In 2015, the Republic of Korea (ROK) experienced a public health crisis due to Middle East respiratory syndrome (MERS), which exposed the weaknesses of the national health disaster response system. Since January 2020, the ROK has been experiencing the coronavirus disease 2019 (COVID-19) epidemic caused by a severe acute respiratory syndrome coronavirus-2 (SARS-CoV-2). MERS and COVID-19 share common characteristics: both are emerging novel coronaviruses with a zoonotic origin and can be fatal, particularly in both older adults and people with chronic medical conditions [1,2]; superspreading events (SSEs) form the overall epi-curve of both outbreaks [3]; and both pose global health threats in the absence of therapeutics or vaccines [4,5]. However, SARS-CoV-2 is more difficult to contain than MERS-coronavirus (MERS-CoV) because of faster human-to-human transmission. Thus, it has spread globally and become a pandemic (Table 1) [1,2,6–19].
MERS-CoV has circulated in the Middle East since 2012, but the ROK is the only country outside of the Middle East that has ever become heavily impacted by the virus [20]. The explosive MERS outbreak in May to June of 2015 revealed many issues in the nation’s public health crisis response [21], before its eventual containment within about 2 months. Since then, improvements have been made at different levels and across the public and private health sectors to protect society against threats from emerging infectious diseases (EIDs). Five years after the MERS outbreak, the COVID-19 pandemic occurred. The country was very successful in quickly flattening the epidemic curve at the early phase of the pandemic without the draconian control measures adopted in most countries [22]. The number of new daily COVID-19 cases reached its lowest level (fewer than 10) in late April 2020. After easing restrictions such as strict social distancing in early May, community transmissions with unknown sources of infection and an inflow of cases from foreign countries have continuously occurred. Furthermore, there have been a series of outbreaks at several mass gatherings that have spread to provincial cities. In this review, we review the lessons from the MERS-CoV outbreak that made the ROK’s response to the COVID-19 pandemic different from that of other countries during early 2020. We also discuss the next steps the ROK can take to further improve control of the virus.
MERS OUTBREAK IN THE REPUBLIC OF KOREA, 2015
On 20 May 2015, a 68-year-old Korean man returning from travel in the Middle East was confirmed as the first MERS case in the ROK [23]. Since the identification of the causative agent in Saudi Arabia in September 2012, 98% of MERS cases have occurred in the Middle East [20]. The propagation of MERS cases from the index case was driven mainly by transmission in one of the hospitals where the index case was admitted [23]. The outbreak resulted in a total of 186 confirmed cases and 38 deaths, with the last case confirmed on July 4, 2015, the 46th day after the first confirmation (Fig. 1) [23]. The outbreak and response affected the entire country and had a detrimental impact in various areas. The socioeconomic impact from the outbreak was estimated to be 9 billion US dollars (1 US dollar equals 1,200 Korean won) [24].
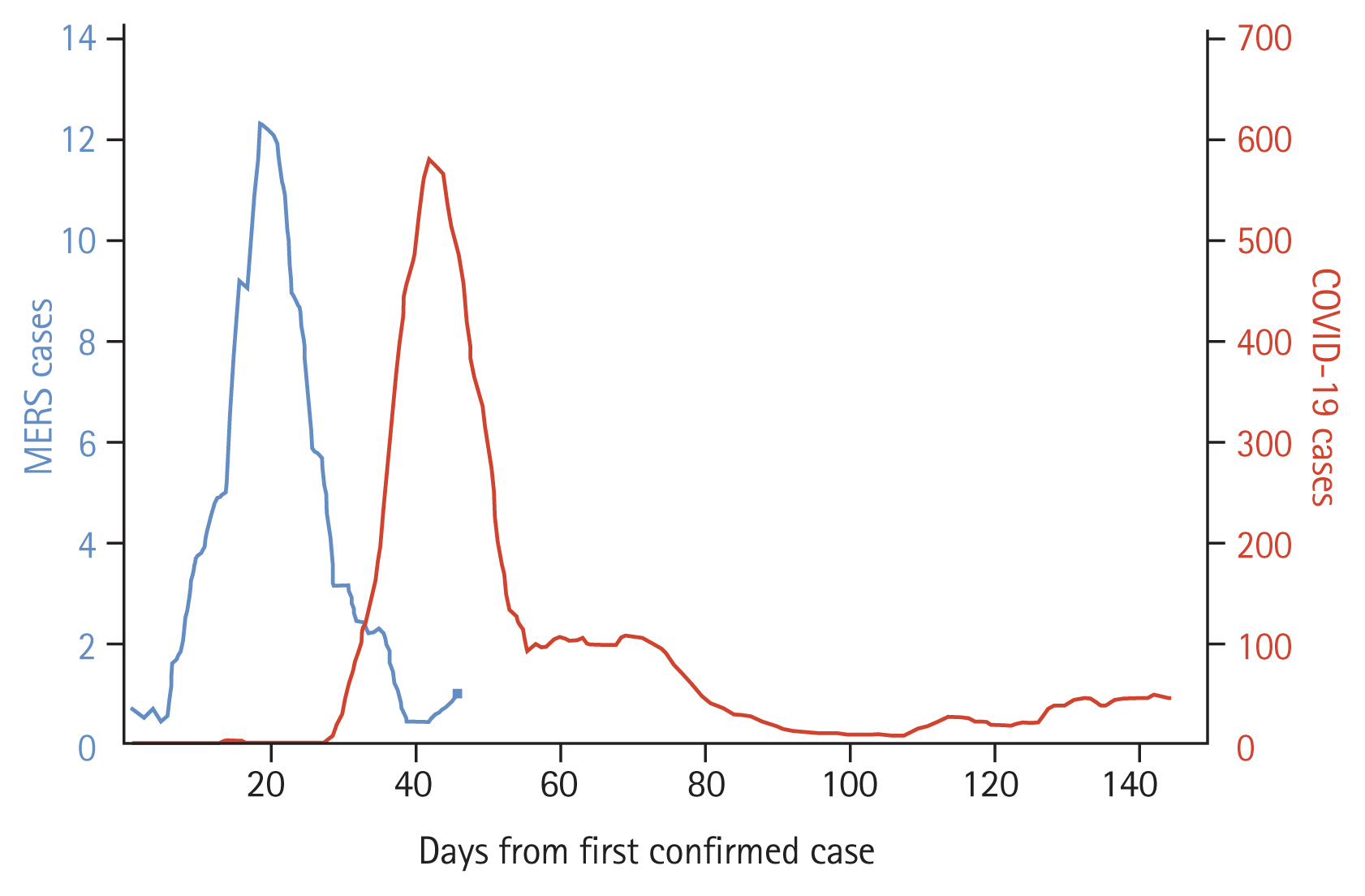
Daily confirmed Middle East respiratory syndrome (MERS, 2015) and coronavirus disease 2019 (COVID-19, 2020) cases in the Republic Korea, rolling 7-day average. The last MERS case in 2015 was confirmed on 4 July 2015, the 46th day after the first confirmed case.
The epidemiology of the MERS outbreak in the ROK in 2015 was characterized by predominantly nosocomial transmissions and hospital clusters related to several SSEs [23,25]. The majority of confirmed cases (93%, 173/186) were infected in healthcare-associated settings (12 hospitals, three clinics, and two ambulances) [25], which is a much higher proportion compared to Middle East countries, where ongoing animal-to-human transmission is occurring [20]. Approximately 80% of transmission events were attributed to five SSEs, which were characterized by more intimate contacts, unprotected visits in crowded emergency rooms or general wards, and “doctor shopping” than non-SSEs [26]. A highly overdispersed pattern of MERS transmission increased the potential for large SSEs, although the overall R0 was less than 1 [27,28].
Characteristics of MERS
MERS is endemic to the Arabian Peninsula, and most cases have occurred in the Kingdom of Saudi Arabia [2]. The natural hosts are thought to be bats, but the virus was transmitted to humans via dromedary camels [2]. Symptoms can range from none to mild or severe, and typically include fever, cough, diarrhea, and shortness of breath [2]. The disease is usually more severe in people with underlying health conditions [2], and its case fatality rate has been described to be as high as 35% [16].
Most MERS cases are symptomatic and the virus is usually transmissible only after symptom onset, aiding control efforts based on isolation of cases and tracing of exposed contacts to interrupt transmission chains [16]. Transmission occurs mainly by respiratory droplet transmission, and airborne transmission has been demonstrated only in healthcare settings where there are aerosol-generating procedures [16]. While sporadic cases of MERS have occurred in endemic regions (probably mostly by direct contact with camels or fresh camel products), larger outbreaks have been confined largely to healthcare settings and caused by nosocomial transmission [2]. Direct evidence for substantial and sustained community transmission remains elusive [2].
2015 MERS outbreak response in the ROK
The ROK was caught off guard by the first MERS cases, but quickly became well-organized and coordinated. Although there was always a high risk for MERS-CoV given the large number of construction workers and businesspeople in the ROK who come from Middle Eastern countries, the MERS cases came as a complete surprise to health authorities. As the number of confirmed cases rapidly increased, stricter outbreak investigation and control measures were implemented, including public disclosure of the affected hospitals, dispatch of a rapid response team, and more robust surveillance and isolation/quarantine policies, between the second and third weeks of the outbreak [21].
The initial epidemic investigation was conducted and the Central Outbreak Control Headquarters was established on the day of case confirmation [21]. The “trace, test, and treat” (3T) strategy was applied in collaboration with ministries, local governments, health experts, epidemic intelligence service (EIS) officers, laboratories, and health facilities. Entry and exit data on international travelers from electronic health registries (the Drug Utilization Review Registry and National Health Insurance Service Beneficiary Registry), Global Positioning System (GPS) systems, and surveillance cameras were used to identify potential contacts with a confirmed case. The integrated MERS information system was established within 2 weeks after the first cases were confirmed. All hospitalized pneumonia cases across the country on June 10, 2015 were screened for MERS-CoV infection via reverse transcription-polymerase chain reaction (RT-PCR) to find undetected cases. A total of 16,693 contacts were quarantined in institutions or at home and monitored by local health authorities over the outbreak period (Table 2). The diagnostic capacity of the laboratory was quickly improved after real-time RT-PCR (rRT-PCR) tests became available in the regional public health and hospital laboratories. The 24 hours MERS hotline (call number 109) also started to operate from May 30, 2015.
The MERS outbreak served as an opportunity for extensive health education to the general public and health workers. Through the repetitive communication of information through multiple channels, the general public learned about the importance of washing hands, wearing a face mask, maintaining respiratory etiquette, and practicing social distancing during the MERS-CoV outbreak [29–31]. Central and local governments, private sectors, and the mass media produced health communication materials. Health workers’ awareness of infection prevention and control (IPC) was improved as well [32]. Approximately 3,000 schools in affected areas were temporarily closed, and a substantial proportion of the Korean population practiced social distancing voluntarily [31].
Challenges
Confirmation of MERS-CoV infection in the index case was delayed by 9 days since his symptom onset. Early detection and response to MERS-CoV were challenging because it was initially mostly unknown to both physicians and the public. However, control measures could have been implemented to minimize contacts and prevent further spread. Furthermore, patients were not routinely asked about their travel history during medical consultations at hospitals or clinics. Given that non-isolated in-hospital days and number of contacts were the most important factors leading to SSEs [25,26], this delay may have played a crucial role in shaping the outbreak.
At the beginning of the response to the outbreak of a novel pathogen, a broader net and more aggressive measures are needed to promptly limit its spread. In the early phase of the MERS response, a narrow case definition of a “close contact” was applied: a person who had physical contact with a confirmed case; or a person who remained within 2 m of a confirmed/suspected case for 1 hour or more while the case was symptomatic. This resulted in many exposed individuals being overlooked, allowing them to transmit the virus beyond the radar of the outbreak investigation [33]. Underestimating the uncertainties about MERS-CoV, such as the high heterogeneity of transmissibility, led to a loose response and a relatively widespread outbreak.
The ROK was insufficiently prepared to detect and respond to EIDs before the MERS outbreak in 2015. There was a lack of functioning EID management systems. The responsibilities and roles of health authorities at the national level were fragmented and lacked clarity [34]. In addition, there was a lack of hierarchical communication between different levels of government, horizontal cooperation, and collaboration between agencies [35]. No public health crisis information-sharing system was initially available, and well-trained human resources were insufficient. EIS officers responsible for investigating the outbreak were over-stretched, as were public health staff charged with contact tracing. There were not enough hospitals with airborne infection isolation rooms (AIIRs) to provide care for all MERS patients. Laboratory diagnostic capacity, which is key to the early detection and control of an EID outbreak, was also constrained [21].
Nosocomial transmission accounted for most MERS cases in the ROK in 2015. The country had hospital-use behaviors predisposing the intra-and inter-hospital spread of emerging pathogens. Doctor shopping, facilitated by existing healthcare systems permitting patients to choose among healthcare practitioners, was identified as one of the risk factors for SSEs [26,36], and enabled a single index case to lead to three generations of secondary infections [37]. The movement of cases between hospitals created a chain of transmission that was difficult for health officials to trace. In addition, families, caregivers, and visitors to hospitals made up 38% (71/186) of the total number of cases in the outbreak. Those who spent time with patients in overcrowded healthcare facilities contributed to the spread of infections [36].
Despite the government’s efforts, the MERS risk communication strategy failed to sufficiently build public trust in the national control measures against MERS [38–41]. Information-sharing with the general public was not sufficiently timely or transparent, and unfounded rumors spread through social media and messaging applications [42]. Misperceptions about disease severity and incidence drove public anxiety and panic. The interests of the government, hospitals, and general public differed sharply with respect to whether the list of affected hospitals should be disclosed [21]. Inefficient inter-agency communication and inconsistent messaging also added to the general confusion.
HOW THE REPUBLIC OF KOREA PREPARED FOR NEW EMERGING INFECTIOUS DISEASES BETWEEN 2015 AND 2020
The ROK learned the hard way from the MERS outbreak. During and after the outbreak, the country developed a national containment infrastructure against EIDs. The master plans for reform of the national infectious disease prevention and management system were announced by the Ministry of Health and Welfare (MOHW) in September 2015, and its implementation was closely tracked [43]. The pillars of the plan included early detection of EIDs, increased surge capacity to handle outbreaks, reform of national infectious disease control systems, and improvement of hospital IPC systems.
The Korean government implemented active monitoring of communicable diseases outbreak situations outside of the country implementing entry screening at airports and seaports, and immediate isolation of suspected cases at a quarantine station in the port of entry [43]. The Emergency Operation Center (EOC) in the Korea Centers for Diseases Control and Prevention (KCDC) was established in January 2016 and has run 24/7 since then. The legislation was amended for a rapid response team to be organized and deployed in case of an incident, and EIS officers are to lead the management of the situation [43]. To enhance the epidemic investigation workforce, the number of central EIS officers was increased from 34 in 2015 to 77 in 2020, and local governments were encouraged to recruit 2 local EIS officers per province [43].
Laboratory capacity was enhanced to accommodate large-scale diagnostic testing in emergency situations. The Korean government has invested in diagnostics research and development, and many private molecular diagnostic companies have also responded. In 2017, the Centre for Laboratory Control of Infectious Diseases in KCDC and the Ministry of Food and Drug Safety adopted the Emergency Use Authorization (EUA) system, which facilitates the rapid approval of diagnostic test kits in response to an EID outbreak [44]. The Center also established a quality assessment program for molecular diagnostics for EIDs in collaboration with the Korean Society for Laboratory Medicine [45].
The KCDC director position was elevated to a Vice-Minister level, and the coordination between central and local governments was improved. The number of regional centers for disease control increased from one in 2015 to five in 2016. The number of staff for infectious disease response at the subnational level increased by more than 360 [46]. Standard operating procedures for crisis management were renewed in June 2016 [46].
During the MERS outbreak in 2015, hospitals learned that insufficient IPC could significantly impact hospital management and finance. They gained institutional knowledge about how to set up MERS-CoV screening stations [47], the importance of proper training for putting on and taking off personal protective equipment, the need for an increasing number of AIIRs, improved room layouts to separate infectious disease patients from non-infectious disease patients, and well-trained healthcare personnel to care for patients with EIDs [48]. Some hospital IPC activities became eligible for financial support from National Health Insurance in July 2016. The Korean Society of Infectious Diseases published the Guidelines on MERS Prevention and Control for Health Facilities in December 2015 [49]. Hospitals were encouraged to limit the number of caregivers and visitors by keeping a logbook or issuing visitor passes. Systems were put in place to encourage hospitals to improve their visitor policies, and those that followed best practices were provided with a certificate [43].
Practices in IPC at emergency departments were improved. More AIIRs and general rooms were created at Emergency Medical Centers [43]. Legislation was introduced in December 2015 requiring Regional Emergency Medical Centers to be equipped with AIIRs [43]. In addition, in 2016, financial support was provided for the establishment and maintenance of AIIRs. The out-of-pocket payment for mild or non-urgent diseases at emergency departments was increased in 2016 to discourage unnecessary visits and reduce crowding. Hospitals were also encouraged to redesign emergency removes to create separate spaces and enable physical distancing between patients.
The 2015 MERS outbreak provided a foundation for amendments to the Infectious Disease Prevention and Control Act that enabled the MOHW and KCDC to collect, and share extensive personal data during serious infectious disease outbreaks [50,51]. In the early stage of the outbreak, detailed movements of individuals were withheld for fear of reidentification and lost business. Later (June 2015), high demand from the public and health professionals justified increased information sharing to alert individuals who may have come into contact with an infected person or contaminated environment [21,33,50].
A joint external evaluation in 2017 concluded that the overall International Health Regulation (2005) core capacities of the ROK were fulfilled, with the majority of indicators in 19 technical areas reflecting a sustainable capacity (60.4%) or demonstrated capacity (31.3%) [52]. It also noted that the country’s impressive preparedness was derived from its recent experience with the MERS outbreak in 2015, and that continued multisectoral effort and stable investment would enable the country to sustain such a high level of capacity.
The country had an opportunity to test and further refine its preparedness soon after the first MERS outbreak. On September 9, 2018, the first MERS-CoV case after the 2015 outbreak was detected in the ROK [46]. The patient, who arrived from the Middle East, visited a hospital on the day he arrived at the airport. The case was immediately confirmed as MERS-CoV infection at the hospital. The government immediately convened a press conference and coordinated a nationwide response. The rapid response team identified 21 close contacts and 487 casual contacts, all of whom were quarantined and monitored. There were no secondary transmissions reported.
COVID-19 EPIDEMIC IN THE REPUBLIC OF KOREA, EARLY 2020
After China, the ROK was one of the first countries to experience a massive surge in COVID-19 cases in the early 2020. The first confirmed COVID-19 case in the country was reported on January 20, 2020; as of June 12, 2020, 12,003 cases and 277 deaths had been reported, with daily new laboratory-confirmed cases peaking at 909 on 28 February 2020 (Fig. 1) [53]. A cluster of cases related to a large religious sect congregation in late February to early March dominated the outbreak, accounting for nearly half of the total number of cases in the ROK (43%). As most cases related to the cluster were young, more than one-third of the total confirmed cases in the entire country (38%) were in their 20s or 30s. As of June 12, 2020, the overall case-fatality rate was 2.3%, ranging from 0 among people under 30 years old to 26% among those aged 80 years or older. Nosocomial transmissions, as well as various community clusters, such as households, nursing homes, call centers, fitness dance classes, churches, and clubs, have been reported [54–56].
Characteristics of COVID-19
As SARS-CoV-2 is a novel virus, rigorous research is ongoing globally and its knowledge is evolving quickly. We have briefly summarized current knowledge about SARS-CoV-2, as of June 2020. SARS-CoV-2, the causative agent for COVID-19 [57], enters its host cell by binding to angiotensin-converting enzyme 2 (ACE2) receptors, which are prevalent on the mucosal surface (Table 1) [58]. SARS-CoV-2 is a member of a large group of related viruses with zoonotic potential (as recognized by World Health Organization [WHO]) carried by bats, which serve as the natural reservoir [59]. The virus is thought to have transmitted to humans through an intermediate host (hypothesized to be pangolins) in a seafood market in Wuhan, China, near the end of 2019. However, this hypothesis has not been verified at present, and other possibilities for the cross-species transmission event have been proposed [60].
SARS-CoV-2 is transmitted between individuals through respiratory droplets and direct contact routes, or by indirect contact with freshly contaminated surfaces [61–63]. Under certain conditions where there is efficient production of respiratory aerosols, the mode of transmission may resemble airborne transmission [64]. SARS-CoV-2 replicates primarily in the upper respiratory tract, but can in some cases cause pneumonia and acute respiratory distress syndrome [12,15]. The virus deserves to be named the “stealth virus,” as it has a relatively long incubation period of around 5 days (2 to 14) [65], which allows it to move silently through populations before being detected [8,66]. In addition, the virus is relatively easily transmitted, probably in both the presymptomatic and early symptomatic stages of infection. It can spread quite rapidly in a population if not recognized quickly [19]. Mass gatherings with high population density and frequent use of elevated voices, particularly in indoor settings with poor ventilation, constitute particularly favorable conditions for outbreaks (e.g., large sports gatherings, religious masses, and concerts) [3]. It is not fully understood whether SSEs are caused by circumstances that are favorable for transmission or by high virus excretion by specific individuals [3].
The majority of those infected suffer a relatively mild or even asymptomatic infection [67]. However, as is the case with many viral respiratory infections, the risk for severe outcomes, including death, increases markedly with age [10]. Male sex, some conditions of the respiratory tract, and immune deficiency are risk factors for severe or fatal outcomes [10]. Estimates of mortality rates are not yet available, but initial estimates are much lower than those described for MERS-CoV, ranging from close to zero in healthy people under 40 years of age to 10s of percentage points in people over 70 years of age with underlying conditions [10].
SARS-CoV-2 is spreading rapidly globally. As of June 13, 2020, 7.7 million cases and nearly half a million deaths had been reported from 216 countries/areas/territories since December 31, 2019, when the outbreak was first reported to the WHO [68]. Effective pharmaceutical interventions, such as vaccines and therapeutics, are not yet available as of June 2020. However, traditional public health containment methods (e.g., early recognition of an outbreak, access to specific test methods such as nucleic acid-based amplification, isolation of cases, and quarantine of exposed contacts) are effective for flattening the epidemic curve [69]. Personal risks can be substantially reduced by carefully maintaining physical distance and rigorous hand hygiene measures [69].
COVID-19 epidemic response in the Republic of Korea
While some countries imposed draconian measures to interrupt the transmission of the virus, including legally restricting the mobility of individuals, the ROK prioritized increasing laboratory test capacity to implement the 3T strategy, which was developed and refined during the previous MERS-CoV outbreaks (Table 2) [70]. KCDC began developing COVID-19 testing on January 13, 2020, when there was only one case reported outside of mainland China. Laboratory testing for COVID-19 first became available at KCDC on January 24, 2020, followed by 17 regional laboratories (Provincial Institutes for Public Health and Environment) [54]. A SARS-CoV-2 rRT-PCR test, for which results are available in 6 hours, became available at all 18 regional laboratories on January 31, 2020 [71] and at private laboratories from February 7, 2020 [54]. By April 2020, five companies’ diagnostic reagents had been granted an EUA, and the number of private laboratories and tertiary hospitals capable of COVID-19 testing had increased to 118 [70]. As of June 12, 2020, the ROK has tested about 1.1 million samples in total, with a maximum daily testing capacity of 60,000. By June 13, 2020, public and private health systems had joined forces to provide laboratory confirmation at 573 testing sites across the country, which has been facilitated by innovative approaches such as drive-through or walk-through testing to meet the massive test demand and ensure the safety of healthcare workers [72,73].
Extensive contact tracing and isolation using information technology (IT) has allowed the government to efficiently break the chains of community transmission. For example, more than 10,000 potential contacts of a single confirmed case who attended a religious sect service were traced and tested regardless of their symptoms. Supported by legislation introduced after the MERS outbreak in 2015, IT enabled efficient tracing. Data from mobile phones, surveillance cameras, and credit transactions have been utilized to identify and quarantine those in contact with the confirmed cases and to quickly isolate those who tested positive [74]. The locations and routes of confirmed cases have been promptly publicized on websites or mobile applications to encourage people to get tested and quarantine themselves if they were in close proximity with any confirmed cases. The IT-supported local authorities monitor symptoms and compliance of contacts and people arriving from other countries under 14-day self- or facility-quarantine. As of April 27, 2020, people who violate the self-quarantine regulation are subject to wear a quarantine tracker wristband or be placed in a quarantine facility at their own cost.
Lives were saved by reserving beds for more severe COVID-19 patients and putting milder cases in dorms. Moderate to severe cases were isolated and treated in AIIRs at government-designated hospitals. Subclinical or mild cases were admitted to community treatment centers (CTCs), which were isolation facilities transformed from dorms, to be monitored and isolated from others [70]. Confirmed COVID-19 cases were treated free-of-charge regardless of nationality. Although the majority of patients were young, the steep increase in cases from the outbreak related to the religious sect congregation strained the healthcare system, particularly in the region where the incident took place. About 20 CTCs were established as of May 2020, and nearly 400 COVID-19-free hospitals have been designated across the country for undisrupted, safe non-COVID-19 clinical practices. In addition, hospitals have been repurposed to increase their capacity to care for COVID-19 patients [75].
Non-pharmaceutical interventions were implemented rigorously in the ROK with the voluntary participation of its citizens. A social distancing campaign started on February 29, 2020; an enhanced phase was put in place on March 22, and restrictions were eased on May 6. The campaign recommended avoiding mass gatherings, postponing the school term from early March to May, and encouraging working from home. A survey conducted in April 2020 indicated that compliance to social distancing practices was as high as 83.4% to 92.3% [31]. Self-hygiene-promotion campaigns were led by both public and private sectors on a large scale. Multiple information channels, including TV and radio spots, posters, and social media, emphasized frequent handwashing, respiratory etiquette, and wearing a mask, which were adopted by three-quarters of the general population [31].
Based on lessons learned from the MERS outbreak in 2015, hospitals proactively enhanced IPC to prevent nosocomial transmission. They quickly established COVID-19 screening stations to prevent non-COVID-19 patients and healthcare workers from being exposed to COVID-19 cases, thereby maintaining routine clinical services. In addition, they implemented strong IPC measures as well as body temperature screening and mandatory mask-wearing. The national guidelines on COVID-19 for hospitals were published on March 10, 2020 [76]. For long-term care facilities (LTCFs), where the most vulnerable populations reside, a designated manager in each facility was responsible for monitoring the response to COVID-19 and communicating with local health authorities. Orientations on IPC were provided to some LTCF staff, and people admitted to LTCF have been screened for COVID-19 prior to admission since May 16, 2020.
The ROK has thus far allowed its borders to remain open. Rather than shutting down borders, the country enhanced screening at all airports and seaports by requiring all people arriving from other countries to fill out questionnaires, monitoring body temperatures, and implementing on-site testing. The Korean government imposed special entry procedures that were expanded from visitors from China (February 4, 2020) to visitors from all countries (March 19, 2020). All international travelers must install a mobile application to report COVID-19-like symptoms daily, as well as their location to the local health authorities, during a mandatory 14-day quarantine.
PREPARATION FOR SUBSEQUENT WAVES
SARS-CoV-2 seems unlikely to follow the course of SARS-CoV, which suddenly disappeared after strong public health countermeasures were implemented across different parts of the world [77]. Instead, it is likely to continue circulating for an extended period of time, causing periodic resurgences depending on factors such as countermeasures, human behavior, and environmental variables [78]. The virus may even persist for some time after a safe and effective vaccine becomes available. In the entire history of mankind, only once has an infectious disease been eliminated by a vaccine. However, in the meantime SARS-CoV-2 may be controlled by self-hygiene, social distancing, and therapeutics.
SARS-CoV-2 was an “unknown unknown”: most governments have struggled to control it in the initial phase. However, the role of government is critical in preparing for subsequent waves of the COVID-19 pandemic. Both central and local governments should develop contingency plans for worst-case scenarios. The pandemic may worsen later in 2020, as COVID-19 and influenza circulate simultaneously [79]. Government agencies should develop strategies to progressively increase healthcare surge capacity to meet peak demand, so-called “raising the bar,” including measures to protect healthcare workers.
Until either vaccine administration or natural infection result in sufficient population immunity, infectious disease control measures should be modified and implemented on a country-specific basis, leveraging the most recent knowledge about the virus to reduce its incidence. These measures include surveillance, quarantine, laboratory testing, case management and isolation, contact tracing, and physical distancing. At the same time, to alleviate response fatigue in the population due to the prolonged emergency situation, the government should carefully select the most appropriate and flexible COVID-19 response activities.
The ROK attracted global attention for its early surge in COVID-19 cases in early 2020, and later for its aggressive interventions (the Korean 3T strategy), which other countries were skeptical about for their perceived non-sustainability and breach of human rights. Rigorous testing, the first element of the strategy, is based on the maintenance of a large number of testing facilities and high testing capacity. The financial sustainability of the strategy and its cost-benefit ratio require further review. Extensive IT-supported epidemic investigation and tracing, followed by strict restriction of mobility for every case and contact, are considered additional elements of the 3T strategy to interrupt transmission. However, human rights controversies have been raised, particularly by Western countries, because such investigation requires the collection of extensive personal medical and nonmedical data [51], and GPS and quarantine tracker wristbands are used to limit the movement of contacts. Broader stakeholder discussions are needed to reach an agreement on the balance between the effectiveness of the interventions and the protection of human privacy rights. Finally, although CTCs have helped reduce the burden of COVID-19, the facility isolation/treatment policy has put a heavy workload on healthcare workers. Because SARS-CoV-2 is transmitted relatively silently and rapidly, and a substantial proportion of COVID-19 cases among vulnerable people can become critical, healthcare systems may be quickly overburdened in the absence of appropriate control measures. Therefore, urgent actions are needed to improve IPC practices in nursing homes and LTCFs [80], repurpose and reshape hospitals [75], increase critical care capacity, and stockpile enough personal protective equipment for healthcare workers. Strategies to prevent burnout among healthcare workers should be established before subsequent waves to support the healthcare system while implementing the 3T strategy.
As the COVID-19 pandemic evolves, false or misleading information has created unnecessary panic in the population. Some serious incidents related to this “infodemic” have been reported in the ROK. For example, a religious group leader unknowingly inoculated his congregation with SARS-CoV-2 by serially spraying saltwater contaminated with the virus into their mouths [81]. Another example is methanol intoxication after using methanol spray for disinfection in an enclosed room. Most scientists are inexperienced in communicating with the public rather than other scientists. To counteract the infodemic, scientists and experts should be proactive in delivering science-based facts and correcting false or misleading information.
FIVE-YEAR VIEW
Although public health preparedness and health system resilience have improved throughout the responses to EIDs in the ROK, further efforts are needed to better prepare for preventing and managing EIDs. To the greatest extent possible, these efforts need to be based on science. Innovation and global solidarity may help with this. One good example is an ongoing project funded by the Gates Foundation utilizing artificial intelligence-based information and communication technology to enhance surveillance and outbreak forecasting [82]. Successful innovations and local experience must be quickly and widely shared at the global level to help overcome common challenges.
Prompt development of diagnostic tests, as well as effective and safe therapeutics and vaccines, is a critical factor for controlling EIDs. Drug repurposing, new drug screening, the use of convalescent plasma, and monoclonal antibody development have been proposed for use as prophylactics or treatments for critical patients [83]. Effective therapeutics may be more easily identified through drug repurposing, which would not require new safety reviews, and therapeutics for very ill require less rigorous safety reviews than vaccines given to large, healthy populations. However, investing in vaccine development remains necessary, because a vaccine is needed to achieve population immunity while avoiding the negative impact of high infection rates [84]. Rapid, mass production of COVID-19 vaccines, distribution of the vaccines to vulnerable or marginalized populations in middle- and low-income countries, low vaccine immunogenicity among older adults, and vaccine safety issues will remain challenges even after vaccines are authorized for use [85].
Rigorous research should be conducted to determine the optimal combination of countermeasures for each country, taking into account sustainability and direct health costs as well as social burden. For example, the health and social benefits of maintaining in-school education should be carefully balanced against the increased risk for SARS-CoV-2 infections. School closures do not only interrupt learning, but may also aggravate inequity and malnutrition for socially disadvantaged children and increase dropout rates, which in turn have long-term economic and social consequences [86]. School closures may create additional child-care obligations [87], and there is not yet robust epidemiological evidence that children are significant drivers of community transmission [88].
The ROK can figuratively be said to have been “vaccinated” against EIDs by the major MERS-CoV outbreak in 2015, which enabled the country to swiftly scale up its EID response systems. Hong Kong, Singapore, and Taiwan, the countries hit hardest by SARS in 2003, responded well to the COVID-19 pandemic during the early phase. They strengthened their EID outbreak response capacity based on lessons learned from past failures, and thereby responded aggressively and promptly to COVID-19 from the beginning. They learned how an unknown infectious disease can disrupt the integrity of society with massive health and economic consequences, and that over-reaction is better than under-reaction.
EIDs will continue to emerge, sometimes with catastrophic economic consequences. Deforestation has resulted in human exposure to various pathogens silently residing in wildlife. Viruses can evolve to infect novel hosts, thus increasing their chances of reproducing. Certain high-risk human activities, such as maintaining live animal markets, increase the likelihood of exposure to animal viruses with zoonotic potential, and globalization and massive international traffic have caused more rapid global EID transmission. The ROK, a major hub for international travel and trade, must be comprehensively prepared for future EIDs.
CONCLUSIONS
The 2015 MERS-CoV outbreak in the ROK served as a stress test to expose weaknesses in healthcare systems and infectious disease control and management systems. Significant progress in response to that outbreak enabled a rapid and aggressive response to the COVID-19 pandemic in early 2020 in the country. The massive epidemic curve has been successfully flattened through the cooperation of the population, the medical community, and the government. In general, the public has adhered well to social distancing, wearing masks, and handwashing. Hospitals responded proactively against the epidemic by enhancing IPC and healthworkers worked hard to diagnose and treat COVID-19 patients. The KCDC has coordinated actions from central and local governments to implement the 3T strategy while mobilizing IT, a major legacy of the 2015 MERS outbreak. However, there is currently a window of opportunity to further increase the surveillance system’s vigilance and strengthen healthcare capacities, particularly in critical care. In the middle of 2020, before a potential second wave, we must rapidly develop functional and comprehensive national preparedness and response plans, in parallel with accelerating the development of vaccines and therapeutics.
Notes
Conflict of interest
No potential conflict of interest relevant to this article was reported.