A mild decrease of renal function is related to increased hemoglobin level during 5-year follow-up period
Article information
Abstract
Background/Aims
We analyzed chronological changes in hemoglobin according to renal function changes over a 5-year follow-up period.
Methods
We enrolled 5,266 adults with a glomerular filtration rate (GFR) ≥ 60 mL/min/1.73 m2 at an initial examination at a routine health check-up; a follow-up examination was conducted 5 years later. We categorized the subjects according to GFR ratio (groups 1, 2, and 3, defined as GFRratio ≥ 1.00, 0.75 to 0.99, and < 0.75, respectively).
Results
The mean hemoglobin level in subjects with a GFR of 60 to 74 was higher than in those with a GFR of 75 to 89 or ≥ 90 mL/min/1.73 m2 at the initial examination (all p < 0.001). Among females and males, the frequencies of increased hemoglobin were 46.8% and 40.6% in the GFRratio group 1, 52.4% and 46.1% in group 2, and 59.6% and 52.5% in group 3 over the 5-year period, respectively (all p < 0.001). With multiple logistic regression, group 3 showed 1.594-fold (95% confidence interval [CI], 1.127 to 2.225) and 1.353-fold (95% CI, 1.000 to 1.830) higher likelihoods of increased hemoglobin over the 5-year follow-up period in females and males, respectively. The estimated difference in hemoglobin level was highest in group 3 in both genders. These findings were more evident in subgroups without metabolic syndrome, diabetes mellitus, hypertension, or GFR less than 90 mL/min/1.73 m2.
Conclusions
Among a population with GFR ≥ 60 mL/min/1.73 m2, a mild decrease in GFR over a 5-year follow-up period was associated with an increase in hemoglobin levels.
INTRODUCTION
Patients with advanced chronic kidney disease (CKD) show decreased hemoglobin levels, primarily because of reduced renal production of erythropoietin (EPO). The National Health and Nutrition Examinational Survey III in the United States reported that the prevalence of anemia was increased in subjects with a glomerular filtration rate (GFR) < 60 mL/min/1.73 m2 [1]. A cohort study including subjects who had stages 3 to 5 CKD determined that the estimated GFR threshold level to detect complications was 44 mL/min/1.73 m2 [2]. However, few studies have investigated changes in hemoglobin concentration and erythropoiesis with mild renal dysfunction.
Here, the study goals were to examine the effects of a mild decrease in GFR on the erythropoiesis response and to determine how GFR levels were related to changes in hemoglobin. We have previously reported that the hemoglobin concentration was slightly, but significantly, increased by small declines in GFR and by the presence of cardio-renal risk factors, such as hypertension (HTN), diabetes mellitus (DM), and metabolic syndrome, which are related to atherosclerosis in early CKD stages [3]. When GFR starts to decrease, the macula densa cells respond to the reduced luminal delivery of chloride, leading to the release of renin, and angiotensin II is activated by its release [4]. Angiotensin II increases oxygen consumption and decreases the oxygen supply by constricting the vasa recta [5]. As a result, renin-angiotensin system (RAS) activation, due to the decreased GFR, leads to a shortage of oxygen in the kidney.
Cardio-renal risk factors are related to atherosclerosis and renal dysfunction [6]. Atherosclerosis increases oxygen consumption in inflammatory cells and decreases oxygen diffusion to tissues, because of the effects of plaque in thickening blood vessel walls [7] and in inducing tissue hypoxia [8,9]. The red blood cell (RBC) number varies according to the decline in GFR and the presence of metabolic syndrome in subjects with GFR > 50 mL/min/1.73 m2. Subjects with lower RBC numbers showed a higher risk of all-cause mortality during 40 months of mean follow-up in a general population [10].
Based on these findings, we previously postulated that the elevation of hemoglobin and RBC number with mild renal dysfunction is not an incidental phenomenon and that it has clinical significance in subjects with early stage CKD and/or the presence of cardio-renal risk factors [3]. However, our previous report was based on cross-sectional data and did not show chronological changes in hemoglobin according to changes in renal function.
In the present study, we developed a database with follow-up findings in the same population as in our previous study [3]. We analyzed factors related to changes in hemoglobin and the effects of progression of renal dysfunction, as measured by estimated GFR, on hemoglobin changes over a 5-year follow-up period in subjects with GFR ≥ 60 mL/min/1.73 m2.
METHODS
Subjects
The study subjects were 5,266 adults, aged 20 or older, with an estimated GFR ≥ 60 mL/min/1.73 m2 at the initial examination, who had had a voluntary routine health check-up at three hospitals affiliated with Seoul National University College of Medicine from 2003 to 2009 as an initial examination and underwent a follow-up examination at 5 years after the initial examination. The subjects answered questions about smoking and drinking habits, medical history of renal disease, DM, HTN, angina pectoris, acute myocardial infarction and self-medication for DM, HTN, or coronary arterial disease prior to the examination.
This study was conducted in accordance with the Declaration of Helsinki. It was approved by the Seoul National University Bundang Hospital Institutional Review Board (H-1003-095-104).
Measurements and definitions
The subjects arrived at the hospitals after an overnight fast of at least 12 hours, completed the questionnaires, and underwent blood and urine tests. Serum creatinine was measured by the Jaffe reaction with an automatic analyzer (TBA-200FR, Toshiba, Tokyo, Japan) in the three hospitals. Serum creatinine levels were calibrated to an assay traceable to isotope dilution mass spectrometry (IDMS; Roche Diagnostics, Mannheim, Germany). The GFR was calculated using the Modification of Diet in Renal Disease (MDRD) study equation [11]. Proteinuria was determined by a urine dipstick test, using an automated Clinitek Atlas urine analyzer (Siemens Healthcare Diagnostics, Deerfield, IL, USA). Complete blood cell counts, including RBC number, hemoglobin, hematocrit, mean corpuscular volume, mean corpuscular hemoglobin, and mean corpuscular hemoglobin concentration were measured and calculated using a Sysmex XE-2100 Hematology analyzer (Sysmex Co., Kobe, Japan).
HTN was defined as systolic blood pressure of ≥ 140 mmHg, diastolic blood pressure of ≥ 90 mmHg or greater, a self-reported history of HTN, or use of antihypertensive medications. DM was defined as a fasting glucose of ≥ 126 mg/dL, HbA1c of ≥ 6.5%, a self-reported history of DM, or use of hypoglycemic agents. A history of coronary artery disease was defined as a self-reported history of angina or acute myocardial infarction. Body mass index (BMI) was calculated based on weight and height (weight/height2, kg/m2). The components of metabolic syndrome were defined according to the National Cholesterol Education Program Adult Treatment Panel III [12]. Anemia was defined as hemoglobin below 13 g/dL in males and below 12 g/dL in females, according to the World Health Organization criteria [13].
We calculated the following: GFR ratio (GFRratio), defined as the GFR level at the follow-up examination divided by the level at the initial examination, hemoglobin ratio (HbRatio), defined as the hemoglobin level at the follow-up examination divided by the level at the initial examination, and the difference in hemoglobin, defined as the hemoglobin level at initial examination subtracted from the level at the follow-up examination. We classified subjects according to the following variables: GFR groups as GFR ≥ 90, 75-89, and 60-74 mL/min/1.73 m2, GFRratio groups as GFRratio ≥ 1.00, 0.75 to 0.99, and < 0.75, and HbRatio groups as HbRatio < 1.00 and ≥ 1.00.
Statistical analyses
All analyses were performed using the SPSS version 18.0 (IBM Co., Armonk, NY, USA) and were conducted separately by gender. Data are presented as means ± standard deviations for continuous variables and as proportions for categorical variables. Differences in continuous variables were analyzed by one-way analysis of variance (ANOVA) or Student t test according to the number of subgroups, and by Pearson chi-square test for categorical variables. We determined the significance of changes in continuous variables at the follow-up examination versus the initial examination using a paired Student t test. We analyzed independent factors related to the increase in hemoglobin during the follow-up period (HbRatio ≥ 1.00) by multiple logistic regression analysis. Analysis of covariance (ANCOVA) was used to estimate the mean hemoglobin level at the follow-up examination after adjusting for independent factors related to the change in hemoglobin during the follow-up period. A p < 0.05 was considered to indicate statistical significance.
RESULTS
Hemoglobin level at the initial examination according to GFR group
The mean hemoglobin level in subjects with GFRs of 60 to 74 mL/min/1.73 m2 was highest among the three GFR groups. In females and males, mean hemoglobin levels were 13.14 ± 1.12 and 15.39 ± 1.01 g/dL in subjects with GFR ≥ 90 mL/min/1.73 m2, 13.43 ± 1.00 and 15.53 ± 0.97 g/dL in GFR 74 to 89 mL/min/1.73 m2, and 13.50 ± 1.06 and 15.57 ± 1.09 g/dL in GFR 60 to 74 mL/min/1.73 m2, respectively (all p < 0.001 by ANOVA). In each GFR group, the hemoglobin level was lowest in subjects without metabolic syndrome components versus subjects with metabolic syndrome and increased with increasing numbers of metabolic syndrome components in both genders (Fig. 1). These results were similar to those in our previous report [6].
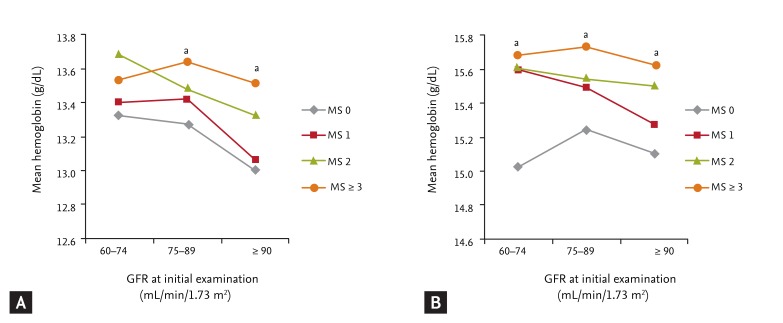
Mean hemoglobin levels at each glomerular filtration rate (GFR) level according to the number of components of metabolic syndrome (MS) in females (A) and in males (B). With increasing numbers of MS components, mean hemoglobin levels tended to increase. ap < 0.05 in the group by number of MS components in each GFR level assessed by one-way analysis of variance. Subjects with no MS component showed lower hemoglobin levels than subjects with MS in females with GFR 75-89 or ≥ 90, and in males with GFR 60-74, 75-89, or ≥ 90 mL/min/1.73 m2.
Clinical characteristics of the GFRratio group
Of the 5,266 identified subjects, 59.0% (3,107 persons) were males. There was no difference in BMI or body weight at the initial examination or the follow-up examination according to GFRratio group in either gender. In females, group 3 (GFRratio < 0.75) showed older age, lower frequency of current smoking, higher levels of fasting glucose, triglycerides, and GFR, and lower levels of cholesterol, HDL-cholesterol, albumin, creatinine, uric acid, and hemoglobin (Table 1). In males, group 3 showed older age, lower frequency of current smoking, higher frequency of DM, higher levels of fasting glucose, and GFR, and lower levels of cholesterol, albumin, creatinine, uric acid, and hemoglobin (Table 1). The frequency of anemia in group 3 was highest in both females (p = 0.009) and males (p = 0.019) (Table 1).
Variables in the initial examination related to increased hemoglobin ratio
We performed multiple logistic regression with variables showing differences in the HbRatio groups and identified independent factors related to the increase in hemoglobin. In females, the relative risks for increased hemoglobin were 0.279 (95% confidence interval [CI], 0.177 to 0.438) for albumin (g/dL), 0.996 (95% CI, 0.993 to 0.999) for cholesterol (mg/dL), 0.463 (95% CI, 0.413 to 0.520) for hemoglobin (g/dL), and 0.727 (95% CI, 0.577 to 0.916) for proteinuria by a dipstick trace test. In males, the relative risks were 0.961 (95% CI, 0.953 to 0.969) for age (years), 0.223 (95% CI, 0.153 to 0.325) for albumin (g/dL), 0.997 (95% CI, 0.995 to 1.000) for cholesterol (mg/dL), 0.994 (95% CI, 0.989 to 0.999) for glucose (mg/dL), 0.565 (95% CI, 0.518 to 616) for hemoglobin (g/dL), and 0.827 (95% CI, 0.688 to 0.994) for proteinuria by a dipstick trace test.
Progression in renal dysfunction is associated with increased hemoglobin over a 5-year follow-up period
In females and males, the frequencies of increased hemoglobin were 46.8% and 40.6% in GFRratio group 1, 52.4% and 46.1% in group 2, and 59.6% and 52.5% in group 3, respectively (all p < 0.001) (Fig. 2). With multiple logistic regression, group 3 showed 1.594-fold (95% CI, 1.127 to 2.225) and 1.353-fold (95% CI, 1.000 to 1.830) higher likelihoods of increased hemoglobin over the 5-year follow-up period in females and males, respectively (Table 2). We estimated the mean HbRatio during the follow-up period using ANCOVA, adjusted with factors related to HbRatio (Fig. 3). The mean HbRatios for females and males were 1.018 (95% CI, 1.009 to 1.028) and 0.995 (95% CI, 0.989 to 1.001) in group 3, 1.007 (95% CI, 1.002 to 1.013) and 0.991 (95% CI, 0.988 to 0.993) in group 2, and 0.996 (95% CI, 0.987 to 1.004) and 0.981 (95% CI, 0.976 to 0.985) in group 1, respectively. The estimated mean difference in hemoglobin was also highest in group 3 in both genders (Fig. 3).
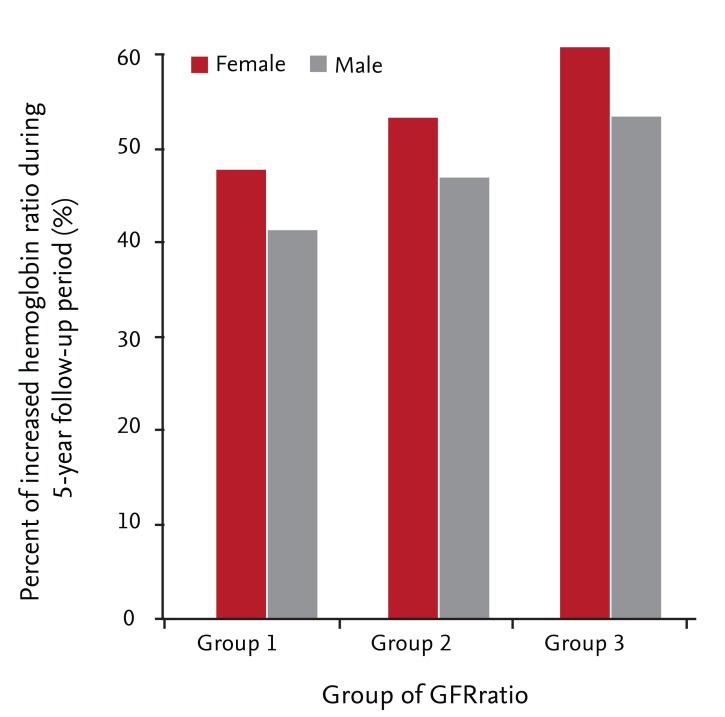
Frequency of increased hemoglobin (hemoglobin ratio ≥ 1) over the 5-year follow-up period by gender. Group 1, GFRratio ≥ 1.00; Group 2, GFRratio 0.75-0.99; Group 3, GFRratio < 0.75, p < 0.001 for females and males. GFR, glomerular filtration rate.
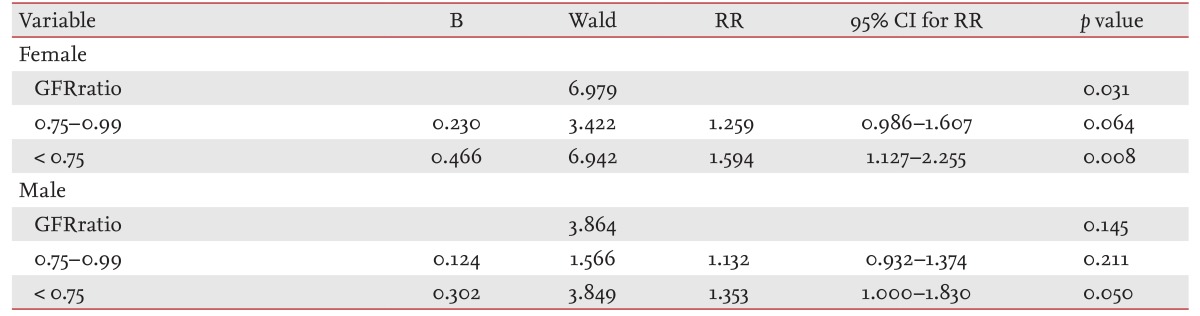
Creatinine ratio group was an independent risk factor for increased hemoglobin over the 5-year follow-up period
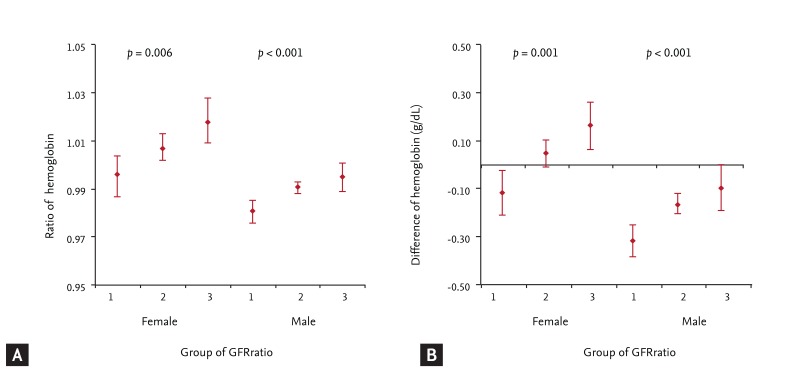
Changes in hemoglobin level measured at the initial examination and at 5 years later according to the change in glomerular filtration rate (GFR) during the same period by gender. (A) Hemoglobin ratio was estimated by covariate analysis adjusted with the factors related to the change in hemoglobin during the 5-year period, such as age, presence of diabetes mellitus and hypertension, systolic blood pressure, diastolic blood pressure, levels of albumin, cholesterol, fasting glucose, triglyceride, creatinine, and urinary protein (dipstick test), and the presence of hepatitis B surface antigen and antibody. (B) Difference between the last hemoglobin and the initial hemoglobin was estimated by covariate analysis adjusted for the factors related to the change in hemoglobin during the 5-year period. A p value indicates the differences between means estimated by covariate analysis. The bar in each column indicates the 95% confidence interval of estimated means. Group 1, GFRratio ≥ 1.00; Group 2, GFRratio 0.75-0.99; Group 3, GFRratio < 0.75.
After stratification of the subjects according to GFR group, DM, HTN, metabolic syndrome, and anemia, we examined the relationship between GFRratio group and the frequency of increased hemoglobin. In the presence of DM, HTN, metabolic syndrome, and anemia, or decreased GFR, less than 90 mL/min/1.73 m2, there was no correlation between GFRratio group and the frequency of increased hemoglobin (Table 3). The decrease in GFRratio correlated with the increase in HbRatio in subjects without DM, HTN, metabolic syndrome, or anemia, or with GFR ≥ 90 mL/min/1.73 m2, in both genders.
Changes in body weight and BMI over the 5-year follow-up period
In females, there was no change in body weight or BMI over the 5-year follow-up period, as estimated using a paired Student t test (56.7 ± 7.0 kg : 56.7 ± 7.0 kg, p = 0.871 for body weight; 22.7 ± 2.8 kg/m2 : 22.7 ± 2.8 kg/m2, p = 0.561 for BMI). In males, body weight and BMI decreased slightly (71.1 ± 9.2 kg : 70.9 ± 9.0 kg, p < 0.001 for body weight; 24.4 ± 2.7 kg/m2 : 24.3 ± 2.7 kg/m2, p = 0.046 for BMI) during the follow-up period. In GFRratio group 3 for males, body weight was unchanged, but BMI decreased slightly (71.1 ± 9.3 kg : 71.1 ± 9.2 kg, p = 0.838 for body weight; 24.4 ± 2.8 kg/m2 : 24.3 ± 2.7 kg/m2, p = 0.032 for BMI). The BMI in group 1 at the follow-up examination had decreased compared with the initial examination, but the BMI in group 2 had not (24.3 ± 2.6 kg/m2 : 24.2 ± 2.6 kg/m2, p = 0.005 for group 1; 24.4 ± 2.8 kg/m2 : 24.3 ± 2.7 kg/m2, p = 0.838 for group 2), and the body weights of groups 1 and 2 at the follow-up examination were decreased versus those at the initial examination (70.7 ± 8.9 kg : 70.3 ± 8.7 kg, p = 0.003 for group 1; 71.1 ± 9.3 kg : 71.1 ± 9.2 kg, p = 0.032 for group 2).
DISCUSSION
Previously, we reported that the hemoglobin concentration increased slightly for subtle declines in renal function and in the presence of cardio-renal risk factors in subjects with GFR > 50 mL/min/1.73 m2 [6]. We postulated that mild renal dysfunction and the presence of cardio-renal risk factors could enhance the RAS and increase renal hypoxia-inducible factor (HIF) and/or EPO in kidneys with normal kidney function or in the early stage of CKD. However, our previous report was based on cross-sectional data and could not show a chronological relationship between changes in hemoglobin and renal function. In the present report, we verified the increase in hemoglobin according to the decrease in renal function and the presence of cardio-renal risk factors in 5 years of follow-up data in subjects with GFR ≥ 60 mL/min/1.73 m2 at the initial examination. Furthermore, in the subgroup analysis, the presence of mild renal dysfunction and cardio-renal risk factors obscured the relationship between the decrease in renal function and the increase in hemoglobin.
Erythropoiesis is regulated by many growth factors, and EPO is required at the late colony forming unit-erythroid stage [14]. The production of EPO is regulated by hypoxia, anemia, or angiotensin II [15]. In a hypoxic state, GATA-2, which suppresses the EPO promoter in normoxia [16], is itself suppressed, causing the activation of HIF and inducing transcription of EPO [17,18]. In humans, plasma EPO concentrations increase exponentially when hemoglobin concentrations fall below 12.5 g/dL in individuals not suffering from renal disease or inflammation [15]. Angiotensin II also stimulates erythropoiesis by increasing EPO production and by acting as a growth factor for myeloid erythrocytic progenitors [5,19]. RAS enhances erythropoiesis, through mainly the angiotensin II type 1 receptor of EPO-producing renal cells, which can be prevented by an angiotensin II type I receptor blocker [20].
In the progression of renal dysfunction in CKD, hypoxia is both a potential cause and an effect of the progression [21]. The loss of postglomerular intertubular capillaries caused by glomerulosclerosis [22], expansion of the interstitial space [23], release of proinflammatory and profibrotic cytokines, increased expression of antiangiogenic factor, and decreased expression of the proangiogenic factor, vascular endothelial growth factor [24], propagate the hypoxia in chronic renal failure. Intrarenal RAS plays an important role in renal autoregulation and pathophysiological developments in renal dysfunction [21]. When GFR starts to decrease, the macula densa cells respond to the reduced luminal delivery of chloride, leading to the release of renin and the activation of angiotensin II [4]. The cardio-renal risk factors are closely related to renal dysfunction [21] and increase the severity of atherosclerosis, which induces hypoxia in the tissue and is caused by hypoxia [5,8,9].
With regard to the regulation of EPO production and the pathophysiological status of CKD, questions remain regarding the time at which the EPO response commences and how it can be detected readily during renal functional deterioration. A few reports have discussed the change in erythropoiesis in populations with normal to mild derangement of renal function. The National Health and Nutrition Examination Survey (NHANES) III in United States [25], data from the Kidney Early Evaluation Program and NHANES 1999 to 2004 [26], and data from the Atherosclerosis Risk in Communities Study [27] showed slight increases in hemoglobin along with declines in GFR, as we report here, but none of these studies examined the relationship in detail. We observed that the decrease in GFR was independently related to the increase in hemoglobin in a population with normal or mildly decreased renal function. The decrease in GFR was clearly related to the increase in hemoglobin in females and, in males, was related to a lesser decrease in hemoglobin. In males, the hemoglobin level decreases with aging. The difference in hemoglobin between males < 30 years old and those ≥ 80 years old is known, 1.49 ± 0.57 g/dL, compared with only 0.20 ± 0.29 mg/dL in females [28,29,30]. Thus, the reduced effect of renal dysfunction on the increased hemoglobin in males versus that in females may be due to the decreased testosterone level during the 5-year follow-up period. The effect of renal dysfunction on erythropoiesis was prominent in a population with normal renal function (GFR ≥ 90 mL/min/1.73 m2) and without cardio-renal risk factors. In our previous study, subjects with mildly decreased renal function (60 mL/min/1.73 m2 < GFR < 90 mL/min/1.73 m2) and with DM, HTN, metabolic syndrome, or anemia showed a pre-existing increase in hemoglobin level compared with subjects without at the initial examination [6] but showed no further increase in hemoglobin with a greater decline in renal function, which is similar to the response to long-term hypoxia. The hypoxia-inducible factor (HIF)-1α level declines during long-term hypoxia, which is related to the induction of the prolyl 4-hydroxylase 3 enzyme by HIF-1α as a functional feedback loop, limiting the hypoxic response [31]. The increase in the intraerythrocytic 2,3-bisphosphoglycerate concentration decreased the O2 affinity of hemoglobin, which may also affect the decrease in EPO in chronic hypoxia [32].
This study had several limitations. First, we used the change in GFR based on serum creatinine as a marker of renal dysfunction, as in other studies. However, the use of creatinine to estimate GFR has limitations because of its tubular secretion and variability in the within-person generation rate [33]. In this population, body weight, which is related to the creatinine generation rate, was unchanged in females and in GFRratio group 3 in males. This reduced the error related to creatinine generation within persons. Use of the CKD epidemiology collaboration (CKD-EPI) equation to estimate GFR has recently been recognized as the most accurate estimation [34]. However, estimated GFR remains limited in its ability to predict true renal function, especially in an older population. Second, the study subjects, who were selected on the basis of data at the follow-up examination, showed characteristics different than those of the whole population that underwent routine health check-ups at our institutions. However, the trend in hemoglobin levels according to the decline in GFR and the presence of cardio-renal risk factors at the initial examination was identical. Thus, the selected population was considered to be representative of the traits of the original population in terms of the relationship between changes in renal function and hemoglobin. Third, we could not exclude nonrenal causes of changes in hemoglobin level, such as iron-deficiency anemia or other hematological diseases. We considered that the large sample size and generally healthy characteristics of the subjects compensated for this. The proportion of anemia was < 10% in females and < 1% in males. Finally, we could not exclude the effects on the results of any medications being taken by the subjects.
In conclusion, the degree of deterioration in renal function was related to the change in hemoglobin levels over the 5-year follow-up period in a population with GFR ≥ 60 mL/min/1.73 m2. This suggests that the kidney responds to the insult in renal function at an earlier stage of renal dysfunction than thought previously. Further studies are needed to identify the pathophysiological mechanism underlying the response to early renal damage and the clinical utility of our findings.
KEY MESSAGE
Even mild renal dysfunction was related to an increase in hemoglobin levels over the 5-year follow-up period in kidneys of normal function or in the early chronic kidney disease stage.
The kidney can respond to an insult in renal function at an earlier stage of renal dysfunction than thought previously.
Notes
No potential conflict of interest relevant to this article was reported.