Neuroprotective effects of Vitis vinifera extract on prediabetic mice induced by a high-fat diet
Article information
Abstract
Background/Aims
Vitis vinifera grape seed extract (VVE) contains oligomeric proanthocyanidins that show antioxidant and free radical-scavenging activities. We evaluated VVE for its neuroprotective effect in prediabetic mice induce by a high-fat diet (HD).
Methods
Mice were divided into four groups according to VVE dose: those fed a normal diet (ND; n = 10), HD (n = 10), HD with 100 mg/kg VVE (n = 10), and HD with 250 mg/kg VVE (n = 10). After 12 weeks, immunohistochemical analyses were carried out using a polyclonal antibody against antiprotein gene product 9.5 (protein-gene-product, 9.5), and intraepidermal innervation was subsequently quantified as nerve fiber abundance per unit length of epidermis (intraepidermal nerve fiber, IENF/mm).
Results
Daily administration of VVE at doses of 100 or 250 mg/kg for 12 weeks protected HD mice from nerve fiber loss compared to untreated mice, as follows (IENF/mm): controls (40.95 ± 5.40), HD (28.70 ± 6.37), HD with 100 mg/kg (41.14 ± 1.12), and HD with 250 mg/kg (48.98 ± 7.01; p < 0.05, HD with VVE vs. HD).
Conclusions
This study provides scientific support for the therapeutic potential of VVE in peripheral neuropathy in an HD mouse model. Our results suggest that VVE could play a role in the management of peripheral neuropathy, similar to other antioxidants known to be beneficial for diabetic peripheral neuropathy.
INTRODUCTION
Diabetes is a common cause of peripheral neuropathy, and more than half of all diabetic patients suffer from diverse neuropathic symptoms [1]. There are multiple etiologies of peripheral neuropathy. Recently, peripheral neuropathy caused by metabolic syndrome, including prediabetes, has been reported to occur before progression to clinical type 2 diabetes [2,3].
Once diabetic peripheral neuropathy is established, true recovery from nerve damage is difficult and only symptomatic improvement may be expected [4]. Although there are few reports concerning the prevention of peripheral neuropathy in prediabetics, early therapeutic intervention in the course of hyperglycemic nerve injury may be more helpful for the successful management of peripheral neuropathy.
The antioxidant and anti-inflammatory activities of Vitis vinifera grape seed extract (VVE) have been investigated in various diseases [5-7]. Oxidative stress is an important common pathway involved in diabetic neuropathy. Considering the beneficial therapeutic effects of antioxidants, such as alphalipoic acid, on peripheral neuropathy associated with diabetes [8,9], we hypothesized that VVE may also be beneficial for treating peripheral nerve damage in prediabetes or early type 2 diabetes.
In this study, we investigated the effect of VVE on a prediabetic neuropathy mouse model induced by a high-fat diet (HD), by assessing intraepidermal nerve fiber (IENF) density, with the ultimate aim of assessing the therapeutic potential of VVE for peripheral neuropathy.
METHODS
Preparation of VVE
VVE was supplied by Korea Hanlim Co. (Seoul, Korea) and prepared as follows. After collecting ripe grapes, the seeds were separated from the pulp and shade-dried. Then the seeds were crushed into a powder, and the dried, powdered, seed material (1 kg) was extracted with 300 mL distilled water and 200 mL acetone. Then the extract was filtered and concentrated to a volume of 100 to 150 mL. Chloroform was added and the filtrates were pooled and evaporated below 50℃ in a vacuum drier to yield a final preparation of 3.5 g.
Animals
Male 4-week-old C57BL/6J mice were purchased from Charles River Laboratories (Tokyo, Japan) and used as reported previously [10]. Throughout the study, the mice were kept in a pathogen-free animal facility with a 12/12-hour light/dark cycle. The temperature and humidity in the room were maintained at 23℃ ± 1℃ and 53% ± 2%, respectively. The animal care protocol and experimental procedures were approved by the Institutional Animal Care and Use Committee of the Chonbuk National University Medical School.
Study design
For the first week (prestudy), the mice were provided with a pellet diet (Research Diets, New Brunswick, NJ, USA) for circumstantial adaptation. They were randomized into four groups: normal diet (ND), HD, HD with 100 mg/kg VVE (VVE100), and HD with 250 mg/kg VVE (VVE250). For the 12 weeks of the study, mice were fed an experimental AIN-93 modified diet (Table 1). VVE was dissolved in drinking water and administered orally at a regular time once per day. The ND and HD groups without VVE were allowed free access to the same amount of distilled water. Body weight, blood glucose, and food intake were measured at the outset and every following week, and blood glucose levels were measured via tail vein samples using Precision Xtra Plus (Abbott Laboratories, MediSense Products, Bedford, MA, USA). Serum triglyceride and cholesterol levels were measured using a commercial kit (Asan Pharmaceutical Co., Seoul, Korea). After 12 weeks of VVE treatment, an oral glucose tolerance test was performed as follows. After 12 hours of fasting, an oral glucose challenge with 50% glucose fluid (1.0 g/kg) was performed and blood samples (5 µL) were collected from the tail at 0, 30 minutes, and 1, 2, and 3 hours. On the final day of the experiments, mice were anesthetized with ketamine (100 mg/kg) and xylazine (10 mg/kg), and a 3 mm punch skin biopsy was performed on the dorsum of the foot for quantification of innervation.
Peripheral nerve quantification
For immunohistochemical analysis of frozen microtome sections, skin tissues were fixed with periodate-lysine-paraformaldehyde (2% paraformaldehyde, 0.075 M lysine, 0.05 M phosphate buffer, pH 7.4, 0.01 M sodium m-periodate) solution for 24 hours. After thoroughly rinsing in phosphate-buffered saline (PBS) containing 20% glycerol and 0.1 M phosphate buffer for 48 hours at 4℃, tissues were cryoprotected with Tissue-Tec OCT compound (Miles, Elkhart, IN, USA). Sections 40 µm thick were cut perpendicular to the dermis on a sliding microtome (Leica CM 1510, Leica Microsystems AG, Wetzlar, Germany) and immersed in PBS for 15 minutes at room temperature. Then the samples were transferred to microcentrifuge tubes containing Dako Protein Block Serum-Free (Dako, Carpinteria, CA, USA) supplemented with 3% goat serum as blocking buffer. After blocking for 30 minutes on a shaker table at room temperature, sections were washed twice for 10 minutes with PBS and incubated with primary antibody against rabbit protein-gene-product 9.5 (Biogenesis, Poole, UK) at a dilution of 1:100 at 4℃ overnight. The antibodies were diluted in antibody diluent (Dako) supplemented with 1% goat serum. After thorough washing, secondary antibody (goat antirabbit IgG-FITC 1:200, Vector, Peterborough, UK) was loaded for 1 hour at room temperature in a dark room. After washing with PBS as before, sections were placed on slides and mounted with fluorescent mounting medium (Dako). Images were collected and analyzed using a Carl Zeiss Axioskop2 plus microscope (Carl Zeiss, Goettingen, Germany) and Axiovision 5.1 software. All biopsy slides were coded and mixed with a set of normal slides, and examiners were blinded to the code information.
Statistical analysis
Data are presented as means ± SD. One-way ANOVA with a Duncan's post hoc test was used to compare experimental groups. Differences were considered statistically significant if the p value was less than 0.05. Statistical analysis was performed using the SPSS software version 12.0 (SPSS Inc., Chicago, IL, USA).
RESULTS
VVE-dependent weight changes and blood glucose levels in mice
Mice were fed experimental diets (Table 1). Body weight changes according to energy intake were significantly greater in the HD diet groups. However, the weight change in the HD with VVE groups was less than in the HD alone group (Table 2). Serum lipid levels were also significantly different according to diet and administration of VVE (Table 3). However, VVE did not significantly inf luence weight change or lipid lowering, according to ANCOVA results (p > 0.05). Random blood glucose level was significantly increased in the HD group compared to the ND, but there was no significant difference between HD and HD + VVE, regardless of dose (Fig. 1A). Oral glucose challenge tests were carried out after 12 hours of fasting (Fig. 1B). We observed differences in blood glucose levels between the HD groups and the ND group (p < 0.05) but the trend towards VVE-dependent differences was not statistically significant.
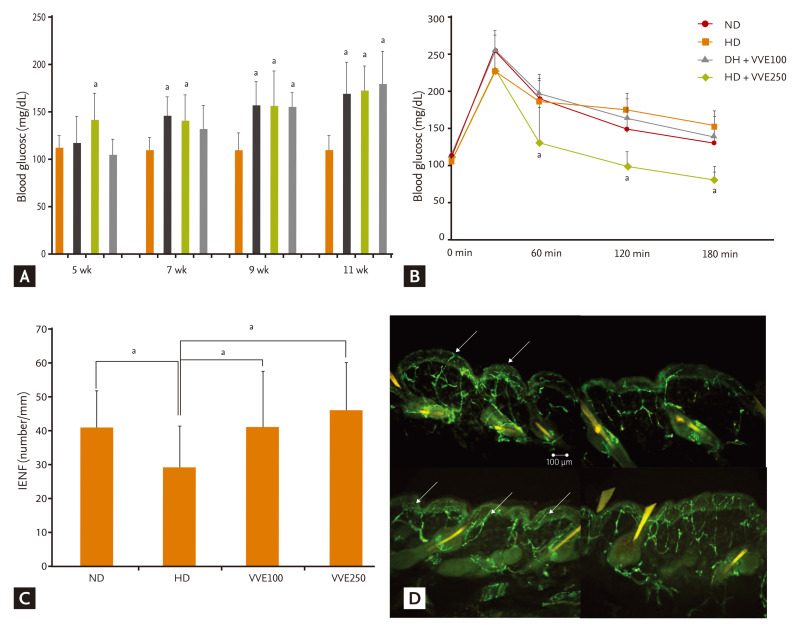
(A) Random blood glucose levels during the experimental period. Although glucose levels were significantly increased in high-fat diet (HD) mice compared with normal diet (ND) mice, there were no significant differences according to Vitis vinifera extract (VVE) administration in HD mice. (B) Blood glucose levels after oral glucose challenge. At 12 weeks, an oral glucose challenge test was performed after 12 hours fasting. Although glucose levels were significantly increased in HD mice compared to ND mice at 60, 120, and 180 minutes, there were no significant differences according to VVE administration in HD mice. (C) Intraepidermal nerve fiber (IENF) counts according to experimental management. IENF density in the HD group was decreased significantly compared to the ND and VVE administration groups. IENF density showed an increased trend after VVE treatment in the HD group. (D) Morphology of IENFs according to diet and VVE treatment. Nerve fibers increased in the VVE administration group. Arrows indicate the epidermal nerve fiber with branching points inside the epidermis.
ap < 0.05 vs. HD group.
Peripheral nerve quantification in mice according to the administration of VVE
The mean value of IENF density (number/mm) was 40.95 ± 5.40 in the ND mice and 28.70 ± 6.37 in the HD mice (p < 0.05). When VVE was administered, the mean value of IENF density in the HD + VVE mice was significantly higher: HD with VVE 100 mg/kg (41.14 ± 1.12) and HD with VVE 250 mg/kg (48.98 ± 7.01; p < 0.05) (Fig. 1C). The morphological findings of IENF in each group are depicted in Fig. 1D, showing an increased and less degenerated pattern of peripheral nerve fibers in the VVE treatment groups.
DISCUSSION
We found that peripheral nerve damage can occur in prediabetic conditions, and VVE had a beneficial effect on peripheral nerve damage prevention in this state, although further research using VVE needs to be performed for overt type 2 diabetes and for more severe states of peripheral nerve damage by hyperglycemia to support its neuroprotective effect. Peripheral neuropathy is common after several years of sustained hyperglycemia, but 20% of early diabetic patients also have symptoms of peripheral neuropathy despite the absence of other microvascular complications [1]. In addition, obesity or prediabetes can induce subclinical peripheral nerve damage in sensory and motor nerve conduction [11]. Metabolic syndrome, independent of hyperglycemia, may also be important in the pathogenesis of peripheral neuropathy [1]. The fatty acid composition of diets is known to affect the development of type 2 diabetes, and a HD may cause glucose intolerance and insulin resistance [12]. Thus, HDs may be related to peripheral neuropathy.
One hypothesis as to the pathogenesis of peripheral neuropathy is that microvascular ischemia may result in impaired neural function and loss of neurotrophic support, with increased reactive oxygen species and endothelial dysfunction [13,14]. Oxidative stress is an important mechanism in the pathogenesis of microvascular and macrovascular complications in diabetic patients [15]. Oxidative stress from hyperglycemia can cause vascular impairment, leading to endoneurial hypoxia in patients with a defective antioxidant response [16]. Glucose auto-oxidation, advanced glycation end products, and the polyol pathway are involved in this oxidative stress [17]. The exact molecular mechanisms involved in complications of glucose regulation are still not understood, and only strict glycemic control remains effective for attenuation of the chronic complications associated with diabetes. However, diverse evidence has lead to general agreement on the benefits of antioxidant therapy for diabetic complications. Experimental and clinical studies have investigated the effects of various agents on diabetic neuropathy, based on these hypotheses regarding pathogenesis. Most of these agents have shown promising results in animal studies, but no convincing findings have been demonstrated in clinical trials. Active management in the early period or before the establishment of diabetic peripheral neuropathy can be considered another therapeutic approach. In this study, we sought to identify the effects of antioxidants on peripheral nerves in an HD-induced prediabetic state.
Grapes are a popular fruit crop and are already widely used to treat many diseases in the indigenous Indian system of medicine (Ayurveda) [18]. The main components of grapes have been investigated with regard to their diverse and beneficial effects, including antioxidant, antiatherosclerotic, antiulcer, and anticarcinogenic activities [19-22]. In addition, VVE has been reported to reduce capillary permeability and thus decrease swelling and inf lammation [23]. VVE contains oligomeric or polymeric flavonoid-like polyphenolic compounds called proanthocyanidins. Oligomeric proanthocyanidins (OPCs) have strong antioxidant and free radical-scavenging effects against oxidative damage. The resulting antioxidant activity of VVE is exerted through inhibition of proteolytic enzymes, such as collagenase, elastase, hyaluronidase, and β-glucuronidase, all of which are involved in the breakdown of vascular structure [24,25]. In fact, several reports have shown that OPCs play important roles in protecting against oxidative stress in the diabetes-induced condition, and nootropic activity of OPCs has been reported in stressed rats [26-28]. However, the effect of VVE on peripheral nerve damage in diabetes has not been well-studied.
In the present study, dietary supplementation with VVE either inhibited peripheral nerve degeneration or improved peripheral nerve regeneration in induced neuropathy in mice. Our failure to observe a VVE dose-dependent effect may be explained by several reasons. First, VVE may have a limited role in neuropathy. Second, neuropathy may be attributed to multiple causes and may exhibit complex pathogenesis, making complete recovery of peripheral nerves by only one intervention unlikely. In addition, the antioxidant effect of VVE might also be involved in the unrelated improvement in IENF density. However, considering the diverse effects of VVE, additional inf luences on the microvasculature or macrovasculature and metabolic factors are likely to be related to peripheral nerve protection. We did not test ND with VVE in our study, because we were specifically interested in the effect on neuropathy. The HD group was the necessary control and the safety of VVE and its other effects have already been reported in previous studies. To determine the exact mechanism of VVE on neuronal cells besides this morphological observation, further studies targeting molecular or inf lammatory markers and VVE-dependent microcirculatory changes are needed.
Blood glucose levels after glucose challenge were not significantly different in the VVE-treated group versus HD mice. However, previous reports have suggested antidiabetic and antilipid peroxidant activities of VVE [29]. Thus, it is difficult to clarify whether the observed effect of VVE is due to direct nerve protection and nerve regeneration, or indirectly, due to glucose control. The glucose levels in our experimental animals were not especially high and these animals were considered to be prediabetic. Thus, a long-term experiment with overtly hyperglycemic animals might be helpful to confirm the antihyperglycemic effects of VVE. Such an experiment could help identify the role of VVE in protecting peripheral nerves, independent of an effect on glucose control. With the availability of more experimental diabetes models, the putative glucose-lowering effects of VVE could be investigated in the future. Serial skin biopsies in the same experimental animal, and a comparison of metabolic parameters according to VVE treatment, would also be informative.
In summary, we found that, apart from weight gain, biochemical measures such as blood glucose, triglyceride, and total cholesterol were elevated in mice fed a HD compared to normally fed mice. Morphological examination of peripheral nerves revealed more degeneration in HD mice. However, VVE-dependent improvement in peripheral nerve morphology, in addition to lipid profiles as in our previous report [10], was observed.
In conclusion, although the antihyperglycemic and antioxidant effects of VVE are well-known, there have been few studies on the nerve-protective or nerve-regenerative potential of VVE in a neuropathic animal model. Here, we investigated the neuroprotective effects of VVE in HD-induced prediabetic mice by measuring IENF density. We observed increased IENF density with VVE administration, suggesting that long-term consumption of VVE might be beneficial for prediabetic or diabetic patients with peripheral neuropathy. At the same time, this result lends support to the traditional claim for the use of grapes and grape seeds in diverse microvascular and macrovascular diseases.
KEY MESSAGE
1. Multiple and complex mechanisms are involved in the diabetic peripheral neuropathy and this peripheral neuropathy can also occur in the prediabetic condition.
2. Oxidative stresses and diverse inflammatory mediators can be important target in the treatment of diabetic peripheral neuropathy.
3. Vitis vinifera extract which have antioxidative and anti-inflammatory effect can be used for the pathogenic management of diabetic peripheral neuropathy in the future.
Notes
No potential conflict of interest relevant to this article is reported.