The effects of pycnogenol on antioxidant enzymes in a mouse model of ozone exposure
Article information
Abstract
Background/Aims
Ozone is an environmentally reactive oxidant, and pycnogenol is a mixture of flavonoid compounds extracted from pine tree bark that have antioxidant activity. We investigated the effects of pycnogenol on reactive nitrogen species, antioxidant responses, and airway responsiveness in BALB/c mice exposed to ozone.
Methods
Antioxidant levels were determined using high performance liquid chromatography with electrochemical detection. Nitric oxide (NO) metabolites in bronchoalveolar lavage (BAL) fluid from BALB/c mice in filtered air and 2 ppm ozone with pycnogenol pretreatment before ozone exposure (n = 6) were quantified colorimetrically using the Griess reaction.
Results
Uric acid and ascorbic acid concentrations were significantly higher in BAL fluid following pretreatment with pycnogenol, whereas γ-tocopherol concentrations were higher in the ozone exposed group but were similar in the ozone and pycnogenol pretreatment groups. Retinol and γ-tocopherol concentrations tended to increase in the ozone exposure group but were similar in the ozone and pycnogenol pretreatment groups following ozone exposure. Malonylaldehyde concentrations increased in the ozone exposure group but were similar in the ozone and pycnogenol plus ozone groups. The nitrite and total NO metabolite concentrations in BAL fluid, which parallel the in vivo generation of NO in the airways, were significantly greater in the ozone exposed group than the group exposed to filtered air, but decreased with pycnogenol pretreatment.
Conclusions
Pycnogenol may increase levels of antioxidant enzymes and decrease levels of nitrogen species, suggesting that antioxidants minimize the effects of acute ozone exposure via a protective mechanism.
INTRODUCTION
The lung interfaces with the external environment and is frequently exposed to airborne pollutants such as ozone and particulates, and is prone to oxidant-mediated cellular damage [1-7]. The production of reactive nitrogen species (RNS) and reactive oxygen species (ROS) associated with oxidative stress are important factors in lung disease [2]. Ozone, a component of photochemical air pollution, is formed from volatile hydrocarbons, halogenated organics, and oxides of nitrogen in the presence of sunlight [2-7]. Ozone can react directly with unsaturated fatty acids and cell membranes to produce lipid ozonation products, which are small, diffusible, and relatively stable [8]. Ozone also leads to the oxidative modification of surfactant proteins, such as surfactant protein-A, which causes the lung to be more susceptible to lipid peroxidation and inflammation, and results in a reduction in phagocytosis [9]. Exposure of human airway epithelial cells to lipid ozonation products in vitro leads to the activation of eicosanoid metabolism, phospholipases A2, C, and D, and the induction of inflammatory mediators such as interleukin (IL)-6, IL-8, and prostaglandin E2 [10,11].
The dietary supplement pycnogenol is a water-soluble mixture of flavonoid compounds extracted from French maritime pine bark. It is utilized throughout the world as a phytochemical remedy for various diseases ranging from chronic inflammation to circulatory dysfunction. The flavonoids in pycnogenol have antioxidant properties [12,13] and may also act as modulators of metabolic enzymes [14-16] and other cellular functions [12,16,17]. Pycnogenol is a very potent antioxidant for scavenging ROS and RNS [16], has anti-inflammatory effects [18], may have efficient antioxidant activity [19-21], and shows some modulatory effects on the immune system [22].
Acute ozone exposure decreases pulmonary function, increases airway responsiveness, and induces airway inflammation [1-7,23-26]. There may be a common ozone adaptation mechanism that involves the regulation of ascorbic acid in the fluid that lines the lungs [27]. Antioxidant transport contributes to the maintenance of normal airway tone and reactivity under conditions of oxidative stress [28]. In the present study, we evaluated the effects of pycnogenol on ROS, RNS, and the antioxidant responses in BALB/c mice following acute ozone exposure.
METHODS
Animals and ozone exposure
Five- to 6-week-old female BALB/c mice, obtained from Daehan Laboratories (Daejeon, Korea), were maintained on ovalbumin-free diets. The mice were individually housed in rack-mounted stainless steel cages with free access to food and water. The mice housed in whole body exposure chambers were exposed to normal ozone concentrations of 0 (filtered room air) and 2 ppm for 3 hours (n = 6/group). Ozone was generated with Sander Model 50 ozonizers (Sander, Eltze, Germany). The concentration of ozone within the chambers was monitored throughout the exposure with ambient-air ozone motors (Model 49C, Thermo Environmental Instruments Inc., Franklin, MA, USA). Air sampling probes were placed in the breathing zone of the mice. The mean chamber ozone concentration (± SEM) during the 3-hour exposure period was 1.89 ± 0.06 ppm. The temperature and humidity were maintained at constant levels within the chamber. Pycnogenol was purchased from Horphag Research Ltd. (Guernsey, UK). The mice housed in whole body exposure chambers were treated with pycnogenol (100 mg/kg/day) orally for 5 days before ozone exposure. The study protocol was approved by the local research ethics committee of the Soonchunhyang University Bucheon Hospital research board.
Determination of airway responsiveness
An increase in the enhanced pause (Penh) was measured by barometric plethysmography using whole body plethysmography (Buxco, Troy, NY, USA) as an index of airway obstruction, immediately after ozone exposure while the animals were awake and breathing spontaneously [29]. Before taking readings, the box was calibrated by rapid injection of 150-µL air into the main chamber. The pressure differences between the main chamber of the whole body plethysmography-containing animal, and a reference chamber (box pressure signal) were measured. This box pressure signal is caused by changes in volume and resultant pressure in the main chamber during the respiratory cycle of the animal. A pneumotachograph with defined resistance in the wall of the main chamber acted as a low pass filter and allowed thermal compensation. The time constant of the box was determined to be approximately 0.02 seconds. Mice were placed in the main chamber, and baseline readings were taken over 3 minutes and averaged.
Bronchoalveolar lavage (BAL) fluid preparation and analysis
BAL was performed immediately after the last measurement of airway responsiveness. The mice were anesthetized intraperitoneally with 50 mg/kg pentobarbital sodium and were sacrificed by exsanguination from the abdominal aorta. The trachea was cannulated with a polyethylene tube through which the lungs were lavaged three times with 1-mL physiologic saline (4 mL total). The BAL fluid was filtered through a wet 4 × 4 gauze. Trypan blue exclusion for viability and total cell counts were performed. The BAL fluid was centrifuged at 150 ×g for 10 minutes. The pellet was immediately suspended in 4-mL physiological saline, and the total number of cells in the BAL fluid was counted in duplicate with a Neubauer improved hemocytometer. A 100-γL aliquot was centrifuged in a cytocentrifuge (Model 2 Cytospin, Shandon Scientific Co., Pittsburg, PA, USA). Differential cell counts were made from the centrifuged preparations stained with Diff-quick; at least ≥ 500 cells per animal were counted at a × 1,000 magnification using oil immersion.
Determination of antioxidant levels
BAL fluid was assayed for ascorbic acid, uric acid, retinol, γ-tocopherol, and γ-tocopherol. Malondialdehyde (MDA) was measured in lung tissue homogenates. Uric acid and ascorbic acid were determined simultaneously using reverse phase high performance liquid chromatography (HPLC) with electrochemical detection, based on the method of Mudway et al. [30]. HPLC determination of retinol, γ-tocopherol, and γ-tocopherol were based on the method of Bieri et al. [31] and described in detail for BAL fluid [32]. Lipid peroxidation, as a marker of oxidative damage, was determined based on the generation of thiobarbituric acid reactive substances in a 10-minute period and expressed as a concentration of MDA, based on the method of Ohkawa et al. [33]. Briefly, the reaction mixture containing 8% sodium dodecyl sulfate, 20% acetic acid (pH 4.0), and 0.8% thiobarbituric acid, was heated at 90℃ for 60 minutes. After cooling, an n-butanol and pyridine mixture (15:1, v/v) was added and centrifuged at 1,000 ×g for 10 minutes. The absorption of the supernatant was measured at 532 nm at room temperature using 1,1,3,3-tetramethoxypropane as an external standard.
Measurement of nitrite and nitrate production
Nitrite production was quantified colorimetrically after the Griess reaction as described by Greenberg et al. [34]. The BAL fluid supernatant, or a standard (100 µL), was combined with an equal volume of Griess reagent (1% sulfanilamide/0.1% naphthylethyllenedihydrochloride/2.5% phosphoric acid, Sigma Chemical Co., St. Louis, MO, USA) in duplicate in microtiter wells at room temperature. Chromophore absorbance at 540 nm was determined. The nitrite concentration was calculated using sodium nitrite (BDH Chemical Co., Poole, UK) as a standard. To assay nitrate, 200 µL of BAL fluid supernatant, or a standard containing 100-µL 200 mM ammonium formate (including 100 mM HEPES, Sigma Chemical Co.) was reduced to nitrite at 37℃ for 1 hour by adding 100 µL recombinant nitrate reductase (Escherichia coli [ATCC25922], American Type Collection, Rockville, MD, USA), followed by centrifugation to precipitate nonreacting E. coli for 5 minutes, after which the nitrite was quantified as described above.
Statistical analysis
All data were analyzed using SPSS version 14.0 for Windows (SPSS Inc., Chicago, IL, USA). Data are expressed as the means ± SEM. Intergroup comparisons were assessed using the nonparametric Mann-Whitney U test. A p value < 0.05 was considered to indicate statistical significance.
RESULTS
Antioxidant responses and RNS
Concentrations of uric acid and ascorbic acid were significantly higher in BAL fluid following pretreatment with pycnogenol (uric acid, filtered air [4,831.9 ± 1,018.2 nmol/mg protein] vs. ozone [13,120.4 ± 2,798.7 nmol/mg protein] vs. pycnogenol plus ozone [21,139.2 ± 2,033.0 nmol/mg protein]; ascorbic acid [19,770.6 ± 4,551.2 nmol/mg protein] vs. ozone [25,058.3 ± 3,365.8 nmol/mg protein] vs. pycnogenol plus ozone [39,319.0 ± 5,010.0 nmol/mg protein], p < 0.05) (Fig. 1).
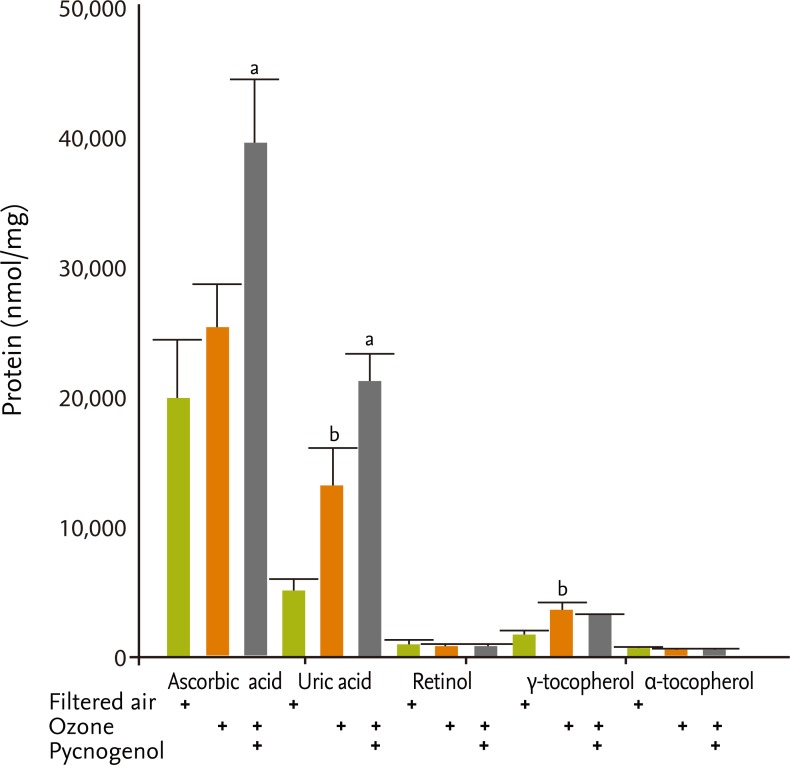
Antioxidant levels in bronchoalveolar lavage fluid. ap < 0.05, compared to the ozone exposure group, bp < 0.05, compared to the filtered air group.
γ-tocopherol concentrations were higher in the ozone exposure group, but were similar in the ozone and pycnogenol pretreatment groups (filtered air [1,572.8 ±248.7 nmol/mg protein] vs. ozone [3,395.6 ± 248.7 nmol/mg protein] vs. pycnogenol plus ozone [3,097.9 ±273.0 nmol/mg protein]) (Fig. 1). Retinol and γ-tocopherol concentrations tended to increase in the ozone exposure group, but were similar in the ozone and pycnogenol pretreatment groups following ozone exposure. MDA concentrations were higher in the ozone exposure group but were similar in the ozone and pycnogerol plus ozone groups (filtered air [2.97 ± 0.08 nmol/mg protein] vs. ozone [3.29 ± 0.19 nmol/mg protein] vs. pycnogenol plus ozone [3.11 ± 0.05 nmol/mg protein]). The concentrations of nitrite and total nitric oxide (NO) metabolites in BAL fluid, which indicate the in vivo generation of NO in airways, were significantly greater in the ozone exposed group than in the group exposed to filtered air (nitrate metabolites in filtered air [40.8 ± 12.9 µmol/L] vs. ozone [98.0 ± 13.9 µmol/L], p < 0.05; total NO metabolites in filtered air [96.5 ± 20.3 µmol/L] vs. ozone [162.9 ± 16.4 µmol/L], p < 0.05), but decreased with pretreatment with pycnogenol (nitrate metabolites, 49.8 ± 11.1 µmol/L; NO metabolites, 116.0 ± 13.4 µmol/L; p < 0.05) (Fig. 2).
Cell counts in BAL fluid and airway responsiveness
The recovery rates of BAL fluid were similar in all groups (2.65 ± 0.03 mL). Compared to that for filtered air, the proportion of neutrophils recovered in BAL fluid increased after exposure to 2 ppm ozone, and there were no differences between the ozone group and pycnogenol plus ozone group (filtered air [1.5% ± 0.21%] vs. ozone [12.10% ± 1.3%] vs. pycnogenol plus ozone [12.15% ± 1.65%], p < 0.01). The increases in Penh after ozone exposure were significantly reduced due to pretreatment with pycnogenol in mice that were exposed to 2 ppm ozone relative to the ozone exposure group (filtered air [0.75 ± 0.10] vs. ozone [1.81 ± 0.07] vs. pycnogenol plus ozone [1.44 ± 0.02]).
DISCUSSION
The results of this study indicate that pycnogenol functions as an antioxidant that reduces airway obstruction following ozone exposure and may modify RNS and antioxidants to minimize the effects of acute ozone exposure.
Oxidative stress is characterized by an imbalance between antioxidant defenses and damaging reactive species. The lungs have an extensive antioxidant network to protect against tissue damage by ROS and RNS [35-43]. ROS and RNS can regulate a diverse array of physiological processes, and deregulation of oxidant signaling may cause or accelerate a host of pathological conditions as it is an important regulator of physiological and pathophysiological outcomes [37]. The lungs have large epithelial surface areas that are exposed to inhaled airborne reactive pollutants and a multitude of airborne microorganisms, which makes them particularly susceptible to environmental oxidant-mediated injury. Metabolic reactions and environmental pollutants such as ozone, cigarette smoke, and particulate matter can produce an oxidizing lung environment, leading to endogenous and exogenous production of ROS [38]. Ozone imposes an oxidative burden on the lung directly as an oxidant during exposure, and indirectly by inducing inflammation. A single, acute exposure to ozone modifies the protective antioxidant defense network in the respiratory tract, with consumption of ascorbic acid and uric acid and reduced glutathione levels [26].
Protective compounds include small antioxidant molecules such as vitamin C, vitamin E, uric acid, the tripeptide glutathione, mucins, metal binding proteins such as transferrin, lactoferrin, and metallothioneins, and a variety of enzymes, such as superoxide dismutases, glutathione dependent enzymes, catalase, and various thiol containing proteins that play an important role in protection against ROS [35-43]. The balance between oxidative burden and the body's antioxidant potential in the pathogenesis of airway obstruction has been the focus of recent investigations. Antioxidants in fluids that coat the lung epithelium constitute an initial line of defense against inhaled environmental oxidants such as ozone, nitrogen oxides, and tobacco smoke. Ozone exposure is associated with adverse respiratory effects, in particular with reductions in lung function [44].
Pycnogenol is a mixture of compounds extracted from the bark of pine trees (Pinus maritima) whose chromatographic profile is composed of phenolic, procyanidin/proanthocyanidin, and flavonoid compounds existing as monomers, dimers, and oligomers of five to seven units [12]. The oligomers are composed mainly of catechin and epicatechin units linked together by four to eight or four to six bonds [12,45]. Other minor constituents of pycnogenol include phenolic acids, glucosides, and glucose esters [12,45]. Pycnogenol lacks toxicity (pharmacologic LD50, 3 g/kg) and is nonteratogenic and nonmutagenic [46]. Pycnogenol is an efficient scavenger of ROS. Indeed, HO· and the superoxide radical anion (O2·-) scavenging activity are maintained after treatment with ascorbate oxidase, indicating that ascorbate, which may be present in the mixture, is responsible for the antioxidant activity. On the other hand, ultrafiltration affects O2·- scavenging activity, suggesting a contribution to the antioxidant activity of high molecular weight compounds present in the mixture [47,48].
Because there are limited data on the effects of pycnogenol and its mechanisms of action, we investigated its effects on RNS, as well as its antioxidant responses and airway responsiveness, following ozone exposure. Our data suggest that pycnogenol has a heightened antioxidant response in mice following ozone exposure. In accordance with previous studies [24,25,44], we found that although the number of neutrophils in BAL did not decrease, increased airway responsiveness following ozone exposure was decreased following pycnogenol treatment after ozone exposure, suggesting that the effect of pycnogenol be not sufficient for inflammatory cells and it could be effective for airway hyperresponsiveness via antioxidant mechanisms.
Antioxidant vitamins are free radical scavengers that can protect against photo oxidant exposure. Vitamins C and E are powerful antioxidants found in the lung that protect against oxidative damage [49]. Although vitamin E is predominantly membrane bound, there is a close interaction between vitamins C and E [50], because vitamin C not only functions directly as an antioxidant but also recycles the antioxidant capacity of oxidized vitamin E [51]. Taking a daily supplement (75 mg vitamin E, 650 mg vitamin C, 15 mg β-carotene) may have a residual protective effect on the lung [52]. Indeed, diet affords protection against ozone induced oxidant toxicity. Protection is mediated partially by increases in ascorbic acid in the fluid bathing the lung surface, thereby providing an antioxidant sink that minimizes the ability of ozone to reach biological targets [53]. In the present study, although uric acid was increased in BAL fluid following ozone exposure and was increased further in BAL fluid following administration of pycnogenol, our data suggest that uric acid may be a protective antioxidant and a marker of the effectiveness of pycnogenol. MDA in BAL fluid is a marker of oxidative damage [32]. In the present study, MDA levels did not change following pycnogenol administration, indicating that MDA may be a less valuable marker of antioxidant effects following ozone exposure.
Few studies have investigated antioxidant levels in BAL fluid in relation to lung function [54,55]. Antioxidant vitamins may play a role in respiratory health; vitamin E and β-cryptoxanthin appear to be stronger correlates of lung function than other antioxidant vitamins. Considerable uncertainty about the association between BAL fluid antioxidants and lung function remains [55]. In the present study, airway responsiveness following pycnogenol treatment was decreased and the uric acid level was increased, suggesting that pycnogenol may increase the antioxidant response and decrease airway obstruction following oxidative ozone exposure. In conclusion, our data suggest that pretreatment with pycnogenol before ozone exposure may mitigate airway obstruction and the oxidizing effects of ozone.
KEY MESSAGE
1. The lungs have an extensive antioxidant network to protect against tissue damage from reactive oxygen and nitrogen species.
2. Ozone imposes an oxidative burden on the lung through direct oxidant exposure and by indirectly inducing inflammation.
3. Pycnogenol may mitigate airway obstruction and the oxidizing effects of ozone.
Acknowledgements
This research was supported by the Korea Ministry of Environment (2012001360001) Environmental Health Action Program.
Notes
No potential conflict of interest relevant to this article is reported.