Erythrocyte Na+-K+ ATPase Activity in the Genesis of Reducing Renal Mass in Hypertensive Rats
Article information
Abstract
To study the relationship between natriuretic factor and experimental low renin hypertension, the erythrocyte Na+-K+ ATPase activities in the genesis of reducing renal mass in hypertensive rats were measured. The results were as follows :
The fractional excretion of Na in hypertensive, reduced renal mass rats (saline-drinking) was higher than that of normotensive, reduced renal mass rats (water-drinking) (p<.001). The plasma renin activities in the hypertensive group were markedly lower than those of the normotensive group (p<.001).
The total ATPase activities of rat erythrocyte membrane in the hypertensive group were lower than in those of the normal and the normotensive groups (p<.001, p<.02).
The Mg++-ATPase activities of rat erythrocyte membrane in the normotensive group tended to decrease significantly (p<.001), but the differences between the normotensive and hypertensive group were not significant.
The Na+-K+ ATPase activities of rat erythrocyte membrane in the hypertensive group were lower than those of the normal and the normotensive group (p<.001). However, the difference between the normal and the normotensive groups was not significant.
When erythrocyte membrane taken from normal rats was incubated with supernates of boiled plasma from normotensive rats, the total ATPase activities of erythrocyte membrane were not different from those of hypertensive rats.
When erythrocyte membrane taken from normal rats was incubated with supernates of boiled plasma from normotensive rats, the Mg++ ATPase activities of erythrocyte membrane were lower than those of hypertensive rats (p<.01).
When erythrocyte membrane taken from normal rats was incubated with supernates of boiled plasma from hypertensive rats, the Na+-K+ ATPase activities of erythrocyte membrane were lower than those of normotensive rats (p<.01).
Based on the above findings, it is suggested that the pathogenesis of low renin, reduced renal mass hypertension is primarily mediated by a natriuretic factor (or an ouabain-like factor, inhibitor of Na+-K+ ATPase) produced by extracellular fluid volume expansion.
INTRODUCTION
Natriuretic hormones (NH) may be divided into two groups on the basis of whether their identity is known or unknown. Some prostaglandins, catecholamines, kinins, insulin, calcitonin, and vasopressin belong to the first group. However, it must be stressed that although the natriuretic action of the hormones listed has been repeatedly documented, the role of the hormones in the physiology or pathophysiology of renal sodium excretion has not been completely clarified.1) Since the initial observations of de Wardener and coworkers2) (1961), many investigators have attempted to determine whether there is indeed a second group consisting of NH of unknown identity.
Biologic effects that have been claimed for the putative NH include (1) natriuresis, (2) inhibition of sodium transport (antinatriferic activity), (3) Na+-K+ ATPase inhibition, and (4) increased vascular reactivity.3)
NH has recently become known as the atrial natriuretic factor (ANF), which is considered to be released from atrial granules and ouabain-like factor (OLF) with no known releasing site. However, there are reports about the isolation of OLF from the hypothalamus4,5) and the kidney in salt loaded rats.6,7) Furthermore, it’s been already known that this OLF can be detected in patients with chronic uremia, those suffering from water immersion, idiopathic hyperaldosteronism, skull injury, high-salt diet and loading, idiopathic edema and during space travel under no gravitation.1,5,7,8)
Ylitalo et al.9) (1979) have reported that if salt is not restricted from the diets of animals which have undergone removal of 70–80% of the renal mass by surgical means, the blood pressure rises. A study carried out by Ylitalo and Gross10) in 1979 indicates that increased blood flow and decreased plasma renin activity in renal mass surgically reduced rats can be observed. Schmidt et al.11) also found in renal mass reduced dogs that, unless salt is restricted in the diet, fractional excretion of Na in each nephron increases and that there might be a substance provoking natriuresis in plasma. On the other hand, in the same animals, with salt restriction none of increased fractional excretion of Na occurred and provoked natriuresis when plasma is injected.
The factors determining the level of the blood pressure are mainly the volume of the extracllular fluid and the changes in peripheral vascular resistance, and the contributing elements are the renal blood volume, the glomerular filtration rate, the ability of the kidneys to excrete Na, plasma renin activity, PG, kallikrein system, and cell membrane Na+ transport defect. Among these elements, the cell membrane Na+ transport defect, that has been studied most, was supposed by Bianchi and Barlassina in 1983 to be a result of inhibited Na+-K+ ATPase activity by OLF, but the correlation between OLF and cell membrane Na+-K+ ATPase activity has not been clarified.12–15)
This study has been carried out for a further investigation of the relationship between the erythrocyte membrane Na+-K+ ATPase and OLF in reduced renal mass, hyporeninemic, hypertensive rats.
MATERIALS AND METHODS
1. Preparation of the Model
Sixty normotensive Wistar rats (150–250 g) were divided into control (n = 14) and experimental groups, and the latter group was again divided into normotensive (n = 23) and hypertensive groups (n = 23).
2. Methods
1) Experimental Group
Control rats drank distilled water and consumed the usual rat chow without subtotal nephrectomy, while experimental rats, under anesthesia with ketamine 25–50 mg/kg I.M., underwent arterial ligation (1/2–2/3) of one kidney and, one week later, the other kidney was removed, reducing the renal mass by 70–80%. Then these experimental animals were fed a low sodium diet (0.02% sodium). Normotensive animals drank distilled water, while hypertensive animals drank a 1% NaCI solution. Systolic blood pressures were monitored for four weeks following the operation, with tail plethysmography (Narco-Biosystem Physiography : MK-IV), and after a similar time period, all the mesurements, such as blood chemistry, urine chemistry and plasma renin activity were done in all experimental animals.
2) Isolation of the Erythrocyte Membranes
Four weeks following the operation, the blood collected from the abdominal aorta with a heparinized syringe, with the animal under ketamine anesthesia was prepared for the isolation of the erythrocyte membranes by the method of Bond and Green16) (1971). All procedures were carried out in an environment below 4°C in temperature. After centrifugation (Beckman : J-6B) at 200 g for 10 min, the plasma and the buffy coat were aspirated. RBCs were then washed 3 times with 0.16 M tris-HCI (pH 6.8) in 6 volumes, and lysed with 1 mM tris-EDTA (pH 7.5) in 5 volumes. The hemolysing solution was added to each in a powerful stream, to mix and lyse the red cells well. The lysed red cells were then allowed to stand for 10 minutes, and ultracentrifuged at 1,800 x g for 20 minutes (Hitachi, p 65). The supernatant layer was discarded and the precipitate was then washed several tims with 1 mM EDTA, 10 mM tris-HCI (pH 7.5) until the membrane preparation became almost whitish in appearance, with the hemoglobin removed from it. These membranes, then, mixed with 10 mM imidazole HCI (pH 7.2), as decribed in Lowry’s report,7) were measured for their protein content and, then, incubated at a temperature of −20°C in preparation for the next procedure.
3) Measurement of the Erythrocyte Na+-K+ ATPase Activity
This procedure was carried out 3 days following the isolation of the erythrocyte membranes. The solution for the reaction contained NaCI 110 mM, KCI 10 mM, MgCI2 4 mM, imidazole HCI 30 mM and, had a protein content of 0.2–0.4 mg/ml. The additional steps were carried out in vitro. The reaction was processed for 30 minutes with the addition of tris-ATP 2 mM, which had been pre-treated for 10 minutes at 37°C in a water bath, as a substrate, and stopped with ice-cold, 15% trichloroacetic acid. After this was centrifuged, the supernatant layer, was measured for its content of free Pi, with a spectrophotometer set at 660 nM, a method used by Fiske and SubbaRow18) (1925). ATPase activity was expressed as the unit of 2 mole Pi/mg, protein hour. Taking value from the standard solution as a total ATPase activity, Na+-K+ ATPase activity can be calculated by subtracting the value for Mg++-ATPase (measured when the Na+-K+ activity is suppressed when ouabain 1 mM is added) from the first value.
4) Plasma Renin Activity
Plasma renin activity was measured in 8 animal each from the normotensive and the hypertensive groups. Three ml of blood was drawn from the abdominal aorta of the anesthesized rats into tubes, each of which contained EDTA. The plasma was separated and then measured by radioimmunoassay using a kit from the Dainabot Company.
5) Blood Chemistry
The plasma concentrations of Na and K were measured by autoanalyzer (Corning Co.) ; that of CI by chlondometer (Corning Co.); those protein, creatinine, BUN, by autoanalyzer (Shimadzu Co.) ; and those of Ca and P by a different autoanalyzer (Abbotte VP. Co.).
6) Urine Chemistry
Rats from each group were left to adjust for 5 days, in a metalic cage, and their urines were collected. The urinary Na and K were measured by flame photometer (Erma Co.) and the urinary creatinine, by autoanalyzer (Shimadzu Co.).
7) Preparation of Plasma Supernates
Plasma was prepared from 6–8 ml of aortic blood. It was collected from each rat into a cooled syringe, and then transferred to a chilled tube containing 0.2 ml of heparin (1,000 u/ml) and centrifuged for 10 minutes at 1,100 x g (Beckman, Model J-6B). The plasma was then allowed to stand at room temperature for 30 min to enhance the generation of the “Na+-K+ pump” inhibiting factor, boiled for 5 min to inactivate proteolytic enzymes and precipitate the denatured proteins, to make it possible to collect the supernates. The supernates were then centrifuged again at (Beckman, LB-70) 35.596 x g for 90 min, collected, and frozen (at −40°C) until assayed for the presence of OLF.
8) Assessment of OLF
In order to test for the presence of OLF in the plasma supernates of both the normotensive and the hypertensive rats, the erythrocyte membranes which had been isolated from the normal Wistar rats were then incubated with the plasma supernates, in the normotensive and in the hypertensive groups and the erythrocyte Na+-K+ ATPase activity was measured.19)
RESULTS
1. General Data Concerning Reduced Renal Mass Rats four Weeks Following Nephectomles (Table 1, Fig. 3)
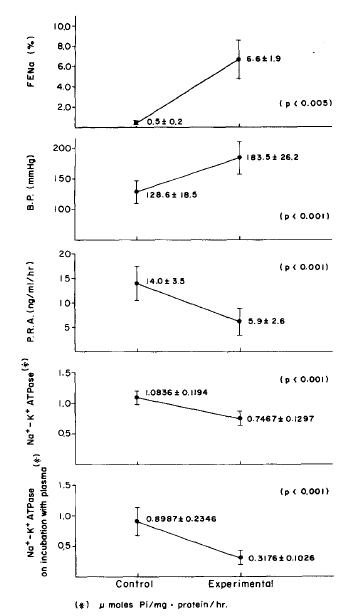
These data show the effect of reduced renal mass hypertension on fractional excretion of Na (FENa), blood pressure (B.P), plasma renin activity (PRA), erythrocyte Na+-K+ ATPase activities and erythrocyte Na+-K+ ATPase activities on incubation with supernates of plasma.
Body weights of the normotensive and hypertensive rats were not significantly different. Systolic blood pressure was greatly elevated in the hypertensive rats which had drunk saline, compared to the normotensive rats which had drunk distilled water (p<.001). The ratio of heart weight to body weight was significantly higher in the hypertensive group compared to the normotensive group (p<.02). Kidney weight was equally increased in the normotensive and hypertensive groups but its ratio to body weight was not significantly different. The plasma renin activity, 14.0±3.5 ng/ml/hr in the normotensive group and 5.9±2.6 ng/ml/hr in the hypertensive group, was significantly different (p<.001).
2. Blood Chemistry of Reduced renal Mass Rats 4 Weeks after their Subtotal Nephrectomies (Table 2)
Serum creatinine was significantly increased in the hypertensive group, while Na, K, CI, BUN, protein, Ca, and P were not.
3. Urine Chemistry of Reduced Renal Mass Rats 4 Weeks after their Subtotal Nephrectomies (Table 3)
Both urine Na and K were significantly increased in the hypertensive group (p<.01, p<.05), while urine creatinine was significantly decreased (p<.05). Particularly, the fractional excretion rate of urine Na, 0.5±0.2% in the normotensive group and 6.6±1.9% in the hypertensive group, showed a magnification factor of about 13 times (p<.005).
4. The erythrocyte ATPase activity in normotensive and hypertensive groups (Table 4, Fig. 1,3)

Erythrocyte ATPase Activity in the Normal, Normotensive and Hypertensive Groups (μ moles Pi/mg. protein/hr.)
The erythrocyte total ATPase activities in the hypertensive group were significantly lowered compared to those of the normotensive group (p<.02), and showed lower values in both groups compared to those in the normal rats which had not had subtotal nephrectomis (p<.001). The erythrocyte Mg++ ATPase activities in both normotensive and hypertensive groups showed no significant difference. The erythrocyte Na+-K+ ATPase activities were significantly lowered in the hypertensive group compared to those of the normotensive group (p<.001), while there was no significant difference between their change in normal rats and the normotensive rats.
5. The erythrocyte ATPase activity on incubation with normotensive and hypertensive plasma (Table 5, Fig. 2,3)
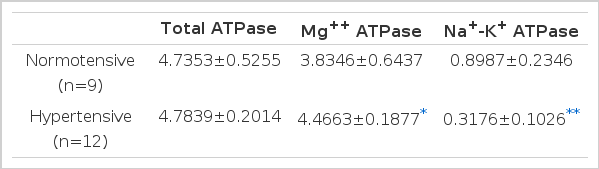
Erythrocyte ATPase Activity on Incubation with Normotensive and Hypertensive Plasma (μ mole, Pi/mg. protein/hr.)
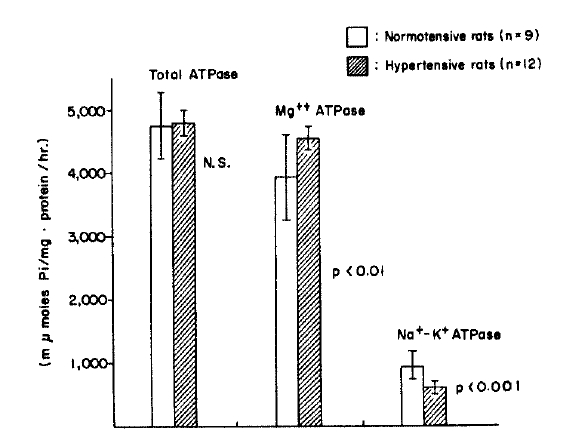
The erythrocyte ATPase activities taken from normal rats when incubated in supernates of boiled plasma from control normotensive and experimental hypertensive rats.
There was no significant difference in the degree of ATPase activity obtained by incubating the supernates of the normotensive rats with the erythrocyte membranes of the normal rats, but the erythrocyte Na+-K+ ATPase activity was greatly lowered when supernates from the hypertensive rats were incubated with 0.3176±0.106 2 moles Pi/mg protein/hr, while those from the normotensive group were incubated with 0.8987±0.2346 2 moles Pi/mg, protein/hr (p<.001). This indicated that the humoral factor inhibiting the erythrocyte Na+-K+ ATPase activity does exist in the plasma, and also that it is heat stable.
DISCUSSION
As the result of the work of Schwartz et al.,20) it has been known that Na+-K+ ATPase, a special enzyme located within the cell membranes of animals, has a sodium pump activity with features of receptor and Na+-K+ exchange. The functional unit for Na+-K+ ATPase has 2 subunits, α and β, which consist of polypeptide chains. Within the inner surface of the α -subunit; (1) receptors for various drugs and hormones, (2) ion-pump function, and (3) ATPase are considered to be present. In other words, β -subunits contain binding sites ; one for cardioactive steroid, one for ATP, three for Na+ ions, and two for K+ ions. Therefore, the action occurs in such a way that 3 moles of Na+ ions are transported out of the cell against its electrochemical and concentration gradients, and this transport is coupled with the active transport of 2 moles of K+ ions into the cell. During the whole process, through the hydrolysis of ATP to ADP providing the energy for transport, a remaining cation outside the cell creates a resting membrane potential.19–22)
Generally, the sensitivity of Na+-K+ ATPase to cardioactive steroid is markedly decreased in the lower animals, including rats, although it is not known this is due to a ouabain-resistance gene or to conversion into ouabain-insensitive Na+-K+ ATPase after the synthesis of the enzyme.22) There are known factors influencing the receptors directly or indirectly. The indirectly controlling factors are (1) ligands of ATP, ADP, Pi, Mg++, and K+, (2) proteolytic enzyme, (3) intracellular enzymes, and (4) genetically produced ouabain controlling protein, and the directly controlling factors are (1) cardioactive steroids, (2) “endogenous factors,” and (3) thyroid hormone, aldosterone, glucocorticoid, catecholamine. Vasodilators, calcium channel blockers, PGE2, vanadium, and dilantin, as exogenous factors, are also directly controlling factors.20,22–24)
So far, the “endogenous factor” has been reported to be isolated from the plasma, urine, kidney, heart, brain, and skin.3,25–27) According to several researchers, “endogenous factor” has been variably known as (1) “endogenous digitalis” (endigin, endoxin), or OLF, (2) natriuretic factors, and (3) “hypertensive factor” isolated from hypertensive patients, and it is not definitely clarified yet whether these are one substance or somewhat modified forms or substances released from different sites.22) Recently, NH has been considered to be divided largely into two groups. One is ANF (or auriculin, cardionatrin, atriopeptin), a peptide consisting of 21–33 amino acids with a molecular weight of 3,000–15,000 dalton, which is released from the atria, but not from the ventricles. Amino acid sequences are defined both in humans (28 a.a.) and rats (21–33 a.a.), but its action mechanism cannot be explained as yet. Even though ANF has a strong effect on both sodium excretion and diuresis and, by depressing vascular resistance, leads to vasodilation, there has been no connection between it and Na+-K+ ATPase activity. Now the interesting topics being focused on are the isolation of antibodies to ANF and the action mechanism.28,29)
The other factor in NH is OLF (circulating sodium pump inhibitor) and this seems to be released from the hypothalamus or the kidney. There are reports of studies done on animals and humans in one of which OLF is described as having a low molecular weight of less than 500 daltons, and in another of which, as having a high molecular weight of 1,500–10,000 daltons.3,30) OLF, which is readily soluble in an organic solvent, and heat-stable, is a peptide digested by prolidase, but not by trypsin and other enzymes. It’s release can be detected by the increase in amount of extracllular fluid such as that which occurs in chronic uremics.31,32) This OLF acts on the renal collecting ducts and tubules and, by inhibiting Na reabsorbtion, its action cause a strongly enhanced Na excretion and diuresis. However, on the other hand, OLF inhibits the efflux of Na from the cell membrane by acting on the erythrocytes, leukocytes, platelets, nerve cells and smooth muscle cells of the vessel. In other words, OLF may partially inhibit the Na+–K+ pump in vascular smooth muscle, thereby causing an increase in norepinephrine secretion from nerve cells, and in intracellular Na+ ion concentration. Then, because the vascular smooth muscle cells contain a Na+ – Ca++ exchange transport system in their plasma membrane, more free calcium ion than normal is delivered to these cells. This caused the in creased vascular tone and peripheral vasaular resistance that elevates the blood pressure.5,15,30,33–36)
It has been known that the NH, play an important role in controlling glomerulotubular balance of Na along with the glomerular filtration rate and the aldosterone. OLF has been detected in chronic uremia, water immersion, primary hyperaldosteronism, head trauma, high salt diet or loading, and idiopathic edematous patients.1,5,7,8) Louis and Favre7) (1980) discovered the existence of OLF in the kidney of the rat that had been rapidly loaded with Ringer’s solution, and they were able to observe its action on the renal collecting duct. Kim et al.37) (1984) also observed the decrease in Na+-K+ ATPase activity of the renal cortex and the increase in the Na+ renal tubular reabsorption, by inhibiting renal tubular Na+-K+ ATPase with the release of OLF from the hypothalamus. This occurs when the circulating blood volume is increased by the rapid infusion of saline or Ringer’s solution.
Welt38) (1969) was able to observe in chronic uremia that the erythrocyte Na+-K+ ATPase activity was markedly decreased. They were also able to observe, when uremic plasma was incubated with erythrocyte membranes prepared from normal subjects, erythrocyte Na+-K+ ATPase activity was markedly decreased. Yoon et al32) (1986) also had observed the markedly decveased erythrocyte Na+-K+ ATPase activity, in chronic dialytic uremic patients. Furthermore, markedly decreased erythrocyte Na+-K+ ATPase activity was observed when the supernates of the heated plasma prepared from chronic uremic patients was incubated with erythrocyte membranes from normal subjects. Although the inhibition of the cell membrane Na+-K+ ATPase in chronic uremia can be considered to be influenced by uremic toxins, such as phenolic acid and methylguanidine, metabolic acidosis, or vanadate within the body, the main point goes to the release of OLF by the accumulation of Na and water in the body.39,41)
However, the inhibition of the erythrocyte Na+-K+ ATPase activity in chronic uremia can recover after renal transplantation. This may be explained by the fact that the activity, inhibited by OLF and other entities is overcompensated following renal transplantation.42,44)
The pathogenesis of essential hypertension has been explained as being a result of interaction among the following factors : (1) genetic or acquired defect of Na excretion ability in the kidney, (2) environmental factors related to age and salt intake, (3) the factor inhibiting Na+-K+ ATPase activity during Na transport across the cell membrane, so called OLF, (4) and others, such as the renin-angiotensin system, catecholamine, the renal nerve, renal PG, and vasopressin. The elevated vascular resistance is due to increased intracellular Ca++ ion concentration, which occurs as a consequence of increased intracellular Na+ and an increased norepinephrine release from the nerve cells by OLF which bring about such an interrelationship as OLF/inhibited Na+ Ca++ exchange/hypertension.13,15,33,45)
In this hyporeninemic, hypertensive group, the increased fractional excretion of urinary Na was 13 times higher than in the normotensive group, the erythrocyte Na+-K+ ATPase activity was decreased, and when heat-treated supernates of the plasma are incubated with the erythrocyte membranes from normal rats, the erythrocyte Na+-K+ ATPase activity is markedly decreased in comparison to that in the normotensive group. It is obvious that a mild uremic state was present in both groups and the possibility that inhibition of the erythrocyte Na+-K+ ATPase activity was due to uremia per se can also be considered. But, as in the hypertensive group, the pathogenesis of hyporeninemic hypertension can be based on the OLF secretion by the mechanism for controlling the Na accumulation following the administration of salt. Then, OLF, by accelerating the excretion of urinary Na and, on the other hand, inhibiting Na+-Ca+ exchange across the cell membranes of the vessel and enhancing norepinephrine secretion from the nerve cells, has explanations from both sides for the elevating of the blood pressure. This can be understood in a way, in that in chronic uremia, as renal function decreases, retention of P causes Ca to be at a low level which, therefore, increases plasma PTH, leading to high urinary excretion of P, this decreasing the plasma P level. But, on the other hand, this is thought to be a result of “trade-off,” a hypothesis explaining the various toxic reactions developed by PTH. In the coming years, the biological and chemical characteristics and secreting sites of OLF should be more throughly investigated and, in order to understand the pathophysiology of hypertension in various diseases, the chemical picture of Na+-Ca++ exchange at the cellular level must be clarified.
Acknowledgements
We are grateful to professor Ku Chul Cho, Mi Yang Whan Chun, Ms Jin Souh Baek for then excellent support.